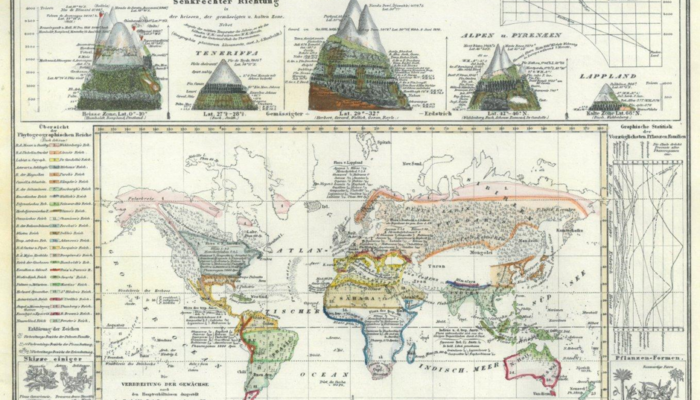
This edition of “Beyond Tectonics” is brought to you by Lydian Boschman. Lydian is a postdoctoral researcher at ETH Zürich. She has a background in geology and plate tectonic reconstructions, but now works with a group of biodiversity modelers of the Landscape Ecology group at ETH, bridging the gap between geology and biology. In her research, she focuses on the uplift history of the Andes, and how mountain building caused species radiations, and ultimately, shaped the modern patterns of biodiversity in South America.
Mountains are perhaps the most tangible expression of plate tectonics to humans. They have inspired many naturalists in the early days of biological and geological science such as Darwin and Humboldt, who observed for example the relationships between altitude, climate and vegetation zones, or how the geographical distribution of wildlife and fossils is tightly linked to the landscape. Most continental mountain ranges are the result of two tectonic plates converging. Orogeny can be collisional (the Alpine-Himalaya mountain range, formed by Africa-Europe and India-Asia collision), or non-collisional (the North and South American Cordilleras, including the Andes, formed above continuous subduction of the oceanic plates of the Pacific Ocean below the North and South American continents).
Mountainous areas are extremely heterogeneous: they contain a much wider variety of types of rock and soil, as well as climate, compared to lowlands. Mountainous areas (especially in the tropics) also happen to be extremely rich in biodiversity: they host roughly 87% of all terrestrial species, while only occupying around 15% of the Earth’s terrestrial surface.
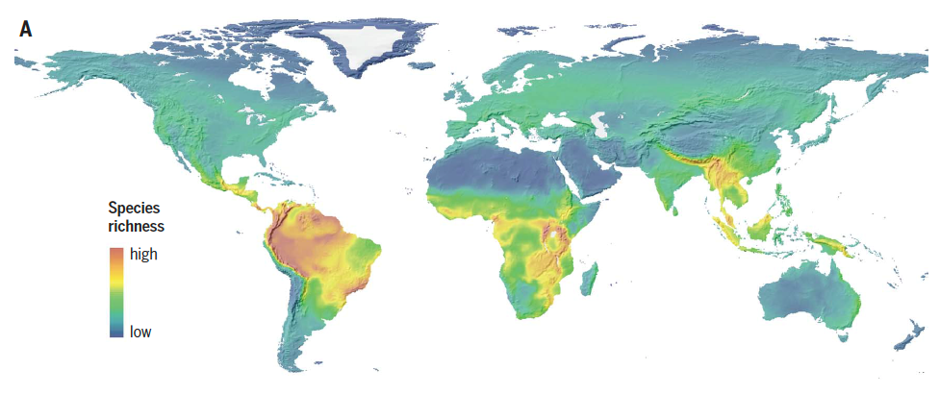
Global richness of vertebrates (birds, mammals and amphibians) and topography. Figure from Rahbek et al. Science, 2019b
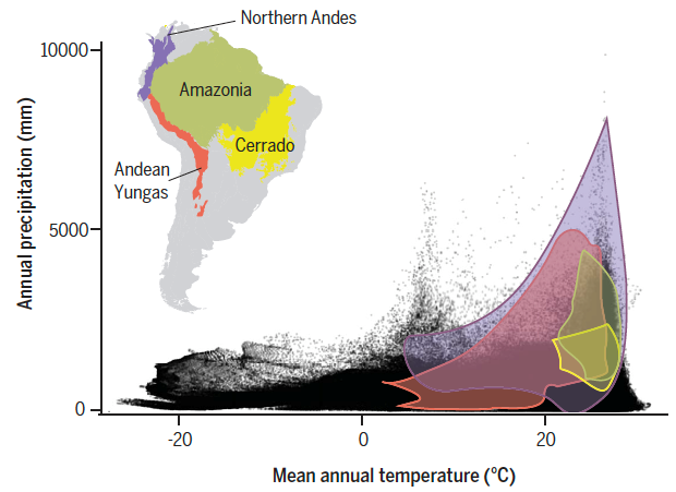
Climate variables of South American regions compared to the total of climate space on Earth (black dots). Note how the mountainous regions contain a much larger climate variability compared to the lowland areas. The Northern Andes are the richest biodiversity hotspot on Earth. Figure from Rahbek et al. Science, 2019b.
Reconstructing mountain building and species radiations
To understand modern patterns in biodiversity, including these sharp gradients in species richness, it is of crucial importance to study them in the context of geology and plate tectonics. To do so, we need reconstructions of horizontal motions of plates over the Earth’s surface, as well as vertical surface motions. Reconstructions of horizontal plate motions provide information on latitudinal motions of the plates and continents, which determines the local climate. Furthermore, these reconstructions constrain when two plates collided or began separating – merging two species pools, or dividing them. Such events are incredibly important in the deep-time evolution of species, but happen relatively rarely. Vertical surface motions, on the other hand, are relevant on a smaller scale. Regional surface motions (uplift or subsidence) continuously change the environmental conditions in, as well as the connectivity (of lack thereof), between habitats. Reconstructing surface motions through geological time is however not straightforward, because the geological record does not contain a direct measure of topography. There are many sources of data that indirectly constrain surface motions, such as low temperature thermochronology, palynology/paleobotany, the identification and dating of paleosurfaces, analyzing the stratigraphic record of foreland basins that quantitatively record the topographic and erosional history of an adjacent mountain range, etc. The most direct measure of paleo-elevation comes from a relatively new method called stable isotope paleo-altimetry (Mulch, 2016; Rowley & Garzione, 2007): values of 𝛿18O and 𝛿D (Deuterium) in the atmosphere vary with elevation, because precipitation from rising air parcels along an orographic barrier removes the heavy isotopes. This means that the 𝛿18O and 𝛿D values in for example paleosols or lakes, fossil teeth, or volcanic glass should in principle record paleo-elevation.
With this set of methods in the paleo-elevation toolbox and more data becoming available every year, it is now more and more possible to make detailed (in both time and space) reconstructions of surface uplift during mountain building. When such a reconstruction is available, it can be compared to a phylogenetic tree of a certain group of species. A phylogenetic tree is a diagram that represents evolutionary relationships between species. Each species included in the tree is represented by a tip, and nodes (where a branch splits in two) represent ancestors. When a phylogenetic tree is dated, for example through fossils, the length of branches and the depth of nodes provides information on when in geological time species evolved. Several recent studies have used this approach of comparing a reconstruction of mountain building with a dated phylogenic tree, and have shown that some species radiations (the splitting of branches, resulting in an increase in taxonomic diversity) in the Andes are directly linked to mountain building (Lagomarsino et al., 2016), whereas in other cases they are more likely to be the result of global climate change during the Pleistocene (Rodríguez-Muñoz et al., 2020).
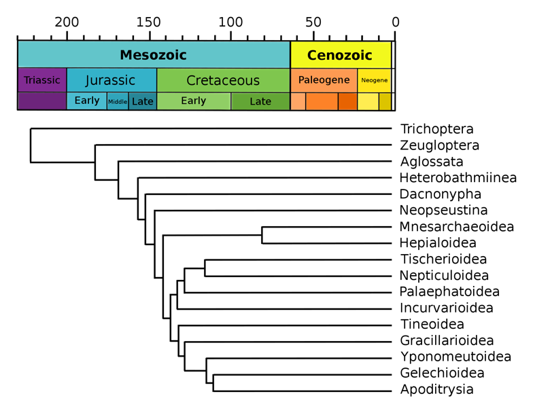
Example of a phylogenetic tree (of Lepidoptera), in which branch lengths are proportional to geological time. Based on Labandeira et al. (1994), Wikipedia.org. Available via licence CC BY-SA 3.0.
Biodiversity modelling
A second new development in the field of combined geo-bio-science is the use of mechanistic models that use tectonic and paleo-elevation reconstructions as well as data on paleo-climate as input, and then compute the dispersion, evolution, speciation and extinction of species in a geographical space. The output of such a model consists of maps of species richness per time, range maps of individual species, and even conceptual phylogenetic trees, which can be compared to empirical biological data sets. A model like this is a great tool for better understanding the underlying mechanisms that play a role in the interaction between geology, climate and biology, because unlike in the real world, modelling allows you to play with parameters and input. This way, it is for example possible to test multiple scenarios for the uplift of the Andes mountains (there is quite a bit of controversy about the uplift of the Central Andean plateau region, see e.g. Ehlers and Poulsen (2009)) and assess what the role of these differences in input is in the resulting biodiversity by keeping all other parameters the same. Similarly, one can test for the role of climate change, or more specifically, the role of the ability of a species to adapt to colder environments (resulting from surface uplift) or warmer environments (due to global climate change), etc. The possibilities are endless, and it seems the coming years will be exciting times for combined geo, climate, and bio-sciences.
Written by Lydian Boschman
Edited by Hannah Davies
Further information:
For an example of a mechanistic biodiversity model, see Rangel et al. (2018). The Rangel model does not include plate tectonics, but only Pleistocene climate change. Our group has been working on a model that does include plate tectonics and goes back much further back in time, which will be published soon. See EGU2020 abstract: “GEN3SIS: An engine for simulating eco-evolutionary processes in the context of plate tectonics and deep-time climate variations”, by Oskar Hagen et al.
For further reading, these are two great papers summarizing the research on moutain diversity since Humboldt’s days:
Rahbek, C., Borregaard, M. K., Antonelli, A., Colwell, R. K., Holt, B. G., Nogues-Bravo, D., Rasmussen, C. M., Richardson, K., Rosing, M. T., & Whittaker, R. J. (2019a). Building mountain biodiversity: Geological and evolutionary processes. Science, 365(6458), 1114-1119.
Rahbek, C., Borregaard, M. K., Colwell, R. K., Dalsgaard, B., Holt, B. G., Morueta-Holme, N., Nogues-Bravo, D., Whittaker, R. J., & Fjeldså, J. (2019b). Humboldt’s enigma: What causes global patterns of mountain biodiversity? Science, 365(6458), 1108-1113.
Other references:
Ehlers, T. A., & Poulsen, C. J. (2009). Influence of Andean uplift on climate and paleoaltimetry estimates. Earth and Planetary Science Letters, 281(3-4), 238-248.
Labandeira, C. C., Dilcher, D. L., Davis, D. R., & Wagner, D. L. (1994). Ninety-seven million years of angiosperm-insect association: paleobiological insights into the meaning of coevolution. Proceedings of the National Academy of Sciences, 91(25), 12278-12282.
Lagomarsino, L. P., Condamine, F. L., Antonelli, A., Mulch, A., & Davis, C. C. (2016). The abiotic and biotic drivers of rapid diversification in A ndean bellflowers (Campanulaceae). New Phytologist, 210(4), 1430-1442.
Mulch, A. (2016). Stable isotope paleoaltimetry and the evolution of landscapes and life. Earth and Planetary Science Letters, 433, 180-191.
Rangel, T. F., Edwards, N. R., Holden, P. B., Diniz-Filho, J. A. F., Gosling, W. D., Coelho, M. T. P., Cassemiro, F. A., Rahbek, C., & Colwell, R. K. (2018). Modeling the ecology and evolution of biodiversity: Biogeographical cradles, museums, and graves. Science, 361(6399), eaar5452.
Rodríguez-Muñoz, E., Montes, C., & Crawford, A. J. (2020). Synthesis of geological and comparative phylogeographic data point to climate, not mountain uplift, as driver of divergence across the Eastern Andean Cordillera. bioRxiv.
Rowley, D. B., & Garzione, C. N. (2007). Stable isotope-based paleoaltimetry. Annu. Rev. Earth Planet. Sci., 35, 463-508.
Associate Professor Subrata Ghosh
Just yesterday (22.08.2020) I attended an excellent online lecture by Dr. Joao C. Duarte (University of Lisbon) on ‘Initiation of plate subduction’. During the question-answer session which followed the lecture, Dr. Joao clearly pointed out the importance of gathering and appreciating broad-based, but critical, knowledge spanning across diverse branches of Earth Sciences (rather than having super-specialised knowledge in a single branch), in order to handle the burning geological issues of global or regional significance properly. The present blog post nicely demonstrated how expertise in biology, geology, tectonics, geography, computer modelling etc. can come together to resolve an important riddle of nature. We anticipate dawning of an age of serious study of various interdisciplinary subjects in Geosciences at the senior UG and/or PG levels in universities across the world.