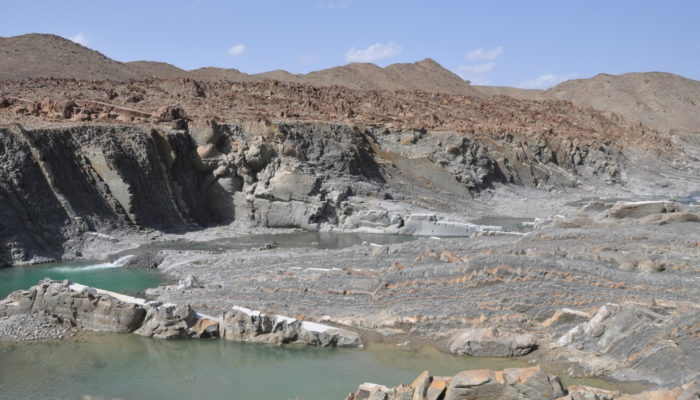
How does an accretionary wedge form?
For this edition of Minds over Methods, we have invited Jonas Ruh, lecturer in the Structural Geology and Tectonics group of the Geological Institute at the Department of Earth Sciences, ETH Zürich, to tell us about the Makran accretionary wedge, one of the largest on Earth. He explains how the use of field observations and numerical modelling helped him to better understand the structural evolution and dynamics of the Makran. Enjoy!
Geodynamic setting
The Makran accretionary wedge is one of the largest on Earth, spanning ~1000 km along, and 350 km across (Fig. 1). It is located in a mostly deserted region in southeast Iran and southwest Pakistan and has not yet been extensively investigated. However, the fact that a large portion of the Makran is exposed on land makes it an ideal case study for accretion processes. Accretionary wedges form along convergent boundaries and denote stacks of sediment sequences scraped off a subducting oceanic plate and accreted onto the overriding plate. The Makran results from the still active northward subduction of the Arabian plate beneath the Eurasian plate that initiated during the Cretaceous (see Burg, 2018 for a review).
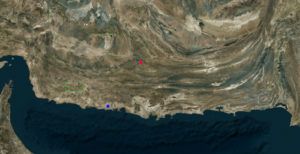
Figure 1: Satellite image of the Makran accretionary wedge striking East–West. Image covers a width of 1100 km. Red dot: Northernmost exposure of colored mélange. Blue dot: Location of mud volcanoes in Fig. 2. Green square: Location of minibasin in Fig. 4. Credit: SAS.Planet.
Geology of the Makran
There are many intriguing geological features characterizing the Makran that may have affected its long-term structural evolution. More than three decades ago, it was suggested that the Makran not only grew by accreting sediments at the wedge front but also at its base, a process called underplating (Platt et al., 1985). This process requires the presence of several mechanically weak horizons, so-called décollements, within the incoming sedimentary sequence, so that the overlaying sediments may scrape off while deeper strata are dragged beneath the wedge. In the Makran, major décollements form due to elevated pore-fluid pressure. In fact, large mud volcanoes along the coast of the Gulf of Oman indicate different stratigraphic levels as mud sources and therefore multiple overpressured potential décollement levels (Fig. 2).
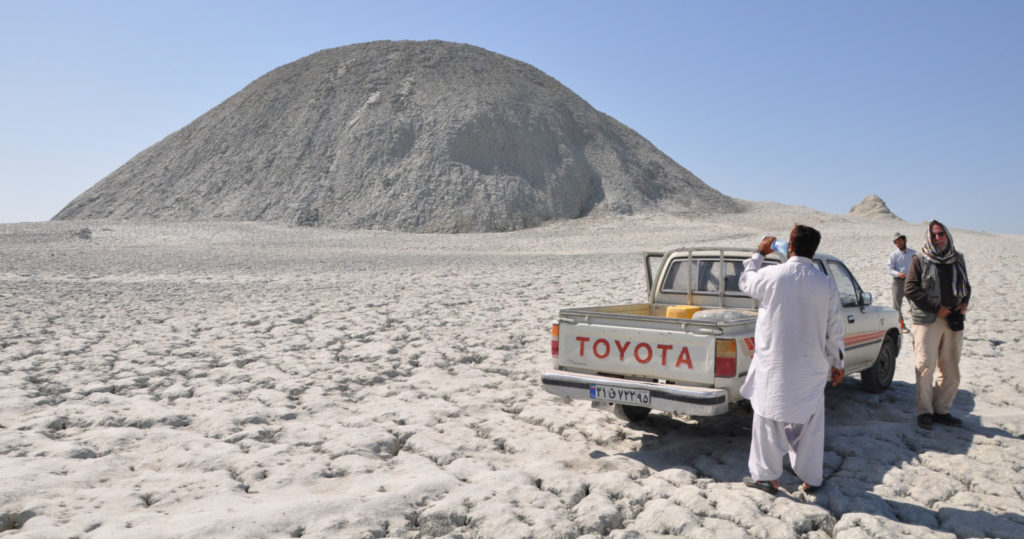
Figure 2: Napag mud volcano west of Chabahar city along the Makran shoreline. Credit: Jonas Ruh.
A huge debris flow covered the accretionary wedge
One of the most outstanding features observed across the onshore Iranian Makran is the occurrence of a “colored mélange”, a mixture of rocks with blocks of variable size and color within a matrix (Fig. 3). This colored mélange has been recently interpreted as a huge debris flow deposited in a submarine environment ~12 million years ago (Burg et al., 2008). Having in mind the age and the northernmost surface exposure of this debris flow (Fig. 1; red dot), it becomes obvious that it must have had a dramatic effect on the structural evolution of the Makran accretionary wedge. Before ~12 million years ago, large parts of today’s entire onshore wedge must have been submarine and, although accreting since the Cretaceous-Paleocene, the wedge front must have been located 100–200 km further to the north.
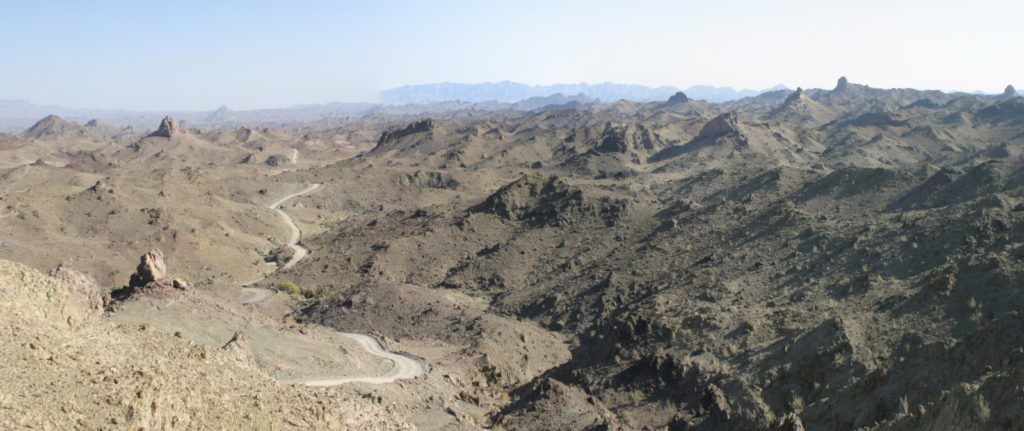
Figure 3: Panoramic view over debris flow exposure. Rigid blocks jutting out of the matrix are well visible. Credit: Jean-Pierre Burg.
Circular folds of the Iranian Makran
Another feature of the onshore Makran are kilometer-wide circular folds (Fig. 4). Satellite imagery reveals that, much like the colored mélange, the spatial distribution of these structures is restricted to the Iranian part of the wedge (Fig. 1). The circular folds are called synclines – folds that open upwards – that formed due to localized sediment accumulation into kilometer-sized depocenters overlaying the yet non-lithified debris flow deposits. The relatively low density and low viscosity of the unsolidified debris flow allowed the accumulating strata in the depocenters to sink into it to form circular synclines as a sedimentary structure without tectonic forcing (Ruh et al., 2018).
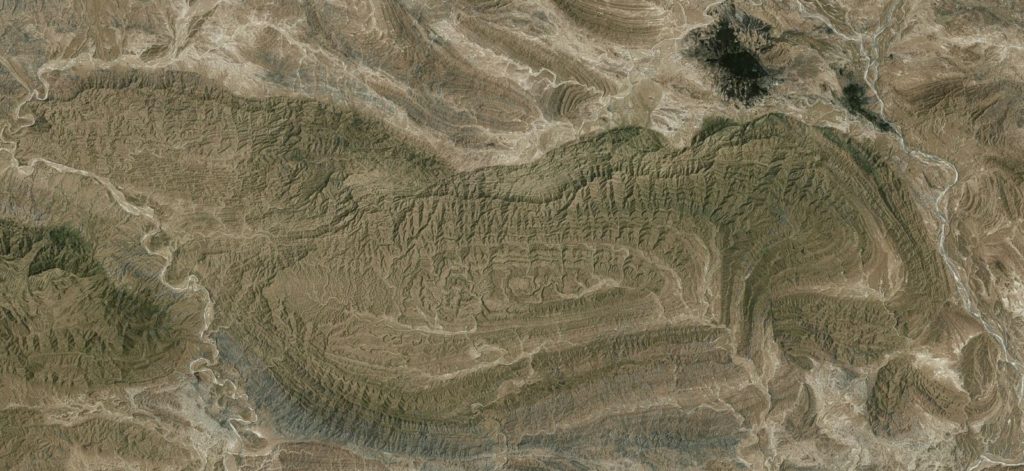
Figure 4: Satellite image of a large circular syncline. Kilometer-size rigid block within the debris flow around the syncline in the top-right. Image width is 70 km. Credit: SAS.Planet.
How did the observed features affect the structural evolution of the Makran?
To answer this question, I set up a numerical experiment with multiple décollements in the incoming sedimentary sequence and the emplacement of a large debris flow with relatively low viscosity and density, after 3 Myr of shortening (Ruh et al., 2018). Before the emplacement of the debris flow, the wedge starts growing where the lower plate is pulled below the backstop (left sidewall) and sediments accrete along two weak décollements (Fig. 5).
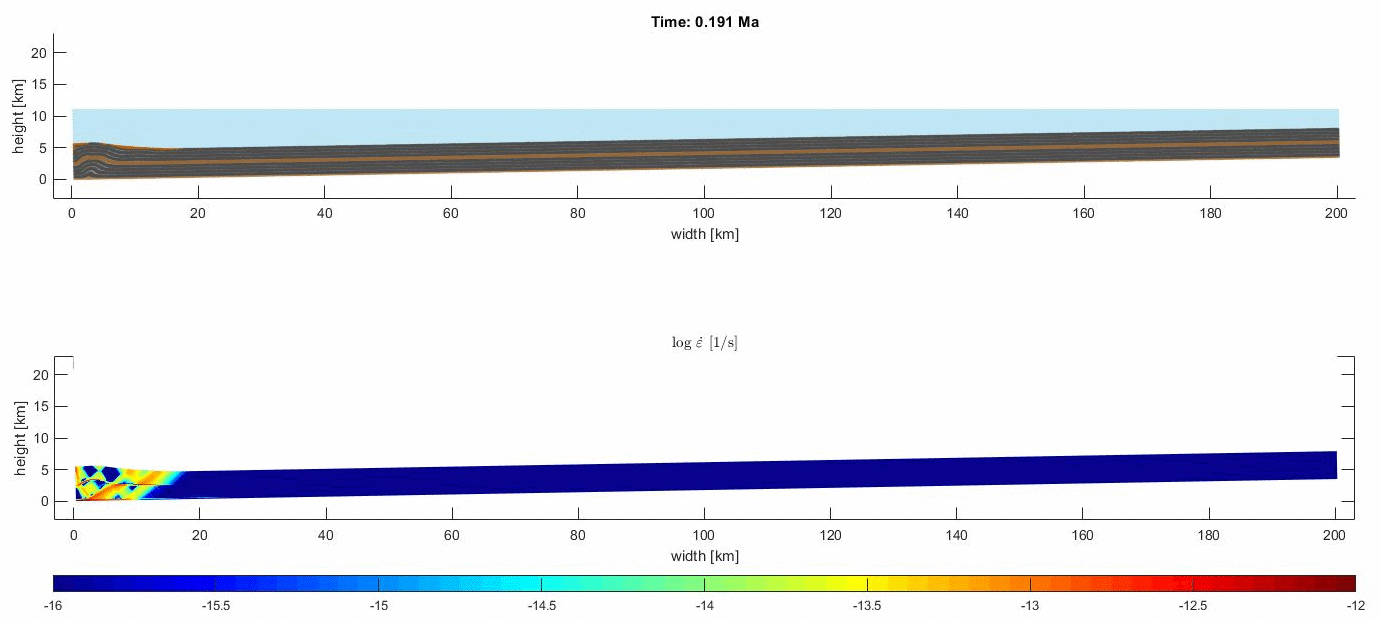
Figure 5: GIF animation of numerical experiment published in Ruh et al. (2018). Top: Rock composition (brown layers: weak décollements; violet colors: post-deris flow; gray colors: incoming sediments). Bottom: Second invariant of the strain-rate tensor where blue indicates slow and red fast velocities. Credits: Jonas Ruh.
The emplacement of the debris flow enhances basal accretion and newly deposited sediments descend into it, forming sedimentary synclines (Fig. 5). Eventually, when the critical taper is sustained, frontal accretion jumps toeward and establishes a new imbricate fan offshore. The onshore part is now characterized by strongly folded and faulted pre-debris flow sequences at the rear and large-wavelength synclines along the shoreline, similar to what is observed in the Makran (Fig. 6).

Figure 6: Numerical experiment after 15 Myr of shortening (Ruh et al., 2018) illustrating main geological features observed in the Makran: underplating, frontal accretion, debris flow and overlying circular synclines.
Outlook
The large-scale numerical experiment is able to reproduce several first-order key features observed in the Makran accretionary wedge such as intense deformation at the rear, the formation of synclines above a large debris flow along the coast, major basal accretion and a newly established frontal imbricate fan (Fig. 6). However, there is still a long way towards fully understanding the Makran. In any case, there will always be a good reason to return to one of the most spectacular and beautiful regions on Earth.
Edited by Silvia Brizzi and Hannah Davies
References
- Burg, J.P., 2018. Geology of the onshore Makran accretionary wedge: Synthesis and tectonic interpretation. Earth-Sci Rev 185, 1210-1231.
- Burg, J.P., Bernoulli, D., Smit, J., Dolati, A., Bahroudi, A., 2008. A giant catastrophic mud-and-debris flow in the Miocene Makran. Terra Nova 20, 188-193.
- Platt, J.P., Leggett, J.K., Young, J., Raza, H., Alam, S., 1985. Large-Scale Sediment Underplating in the Makran Accretionary Prism, Southwest-Pakistan. Geology 13, 507-511.
- Ruh, J.B., Verges, J., Burg, J.P., 2018. Shale-related minibasins atop a massive olistostrome in an active accretionary wedge setting: Two-dimensional numerical modeling applied to the Iranian Makran. Geology 46, 791-794.