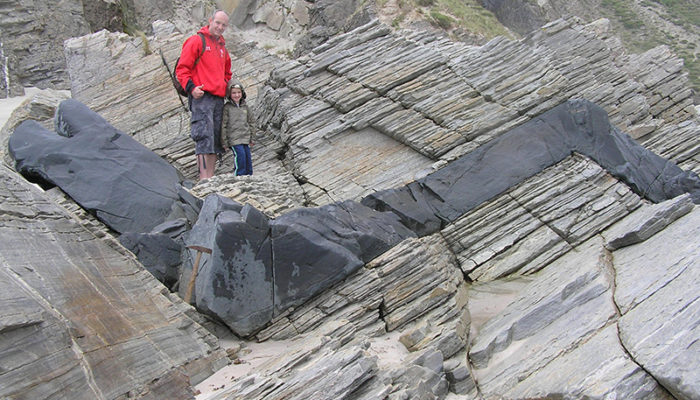
Volcanoes are everywhere along plate margins and on hot spots on the planet’s surface. Just in the past 2 years, we have witnessed enormous explosive eruptions such as that of the Hunga Tonga volcano which released an amount of energy equivalent to hundreds of atomic bombs, and massive lava flows, as at the Cumbre Vieja at La Palma, which was estimated to have a total volume of 80 million cubic meters. And these are just some volcanoes that became popular in the news recently for their sudden eruptions, among the over 1500 volcanoes that are currently active worldwide, like Stromboli and Etna in Italy, the Popocatépetl in Mexico, the Kīlauea in Hawaii, and the Ol Doinyo Lengai in Tanzania, just to name a few.
Volcanic eruptions are spectacular, but they are just the tip of a plumbing system that exists beneath our feet and that links volcanic centers to the site where magma is produced: partially molten rocks tens to hundreds of kilometers deep into the crust and the mantle. These ‘pipes’ efficiently transport hundreds of cubic kilometers of magma beneath island arcs, hot spots, and mid-ocean ridges and not only to volcanoes: they also bring ‘fuel’ to buried magma stocks (plutons) like The Geysers (California, USA) or Larderello (Italy), which we, humans, have learned to harvest for geothermal energy [also check out: Geothermal Energy and Structural Geology], and to more dangerous dormant calderas like Yellowstone (Wyoming), Toba (Indonesia), and the Phlegrean Fields (Italy), were tens of cubic kilometers of magma are currently being stockpiled at depth and may erupt one day.
These pipes aren’t like regular plumbing though, we can’t dig them up and see how they are connected because they are too hot and too deep. We can either view them with geophysical techniques, like ground penetrating radar, or go looking for pipes that plate tectonic collision has “dug up” for us, exposing the pipes beneath volcanoes in mountain belts.
Dikes and sills
When magma forms at depth, it is surrounded by kilometers of solid rock in all directions exerting a high pressure on it. As a less dense liquid under heavier (denser) material, magma tends to ascend within rocks, flowing towards the surface of the planet. The problem is that rocks are quite… solid, and not easy to pass through. Luckily for the magma, rocks have layers of weakness like beds, foliations, faults, and fractures that can be exploited to move across the crust. Magma also moves upwards squeezed by tectonic forces or pushed upwards by the difference in density with the surrounding rocks and, in these cases, it is able to break rocks apart intruding in newly-formed fractures.
Dikes (or dykes) are the most efficient way to transport magma upward within the crust. Dikes are intrusions that are generally near-vertical and that cut through pre-existing bedding planes or foliations within rocks. Dikes propagate by fracturing the surrounding rock and opening a path along the direction of minimum tectonic stress, often assisted by the difference in density between the rocks and the magma, driving magma upward.
Sills, on the other hand form when magma exploits pre-existing weaknesses, such as strata, foliations, or fractures to emplace within rocks. Frequently cited examples of sills are nearly horizontal intrusions that are parallel to the horizontal beds of the surrounding rocks, but actually sill can born inclined, if they exploit inclined mechanical discontinuities (Walker, 1993; Menand, 2011).
Both types of intrusions are always younger than the rocks they intrude.
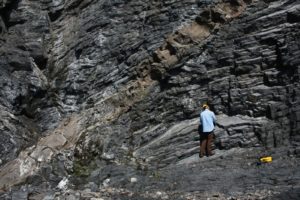
The brownish ‘layer’ dipping to the left and crosscutting this Ordovician sedimentary sequence is a Mesozoic igneous dyke, part of a swarm of hundreds of similar dykes in the region that were associated with the opening of the Atlantic Ocean, which separated North America from Europe. Leading Tickles, Newfoundland, Canada. Photo © Dr. Alexander Peace via Imaggeo.
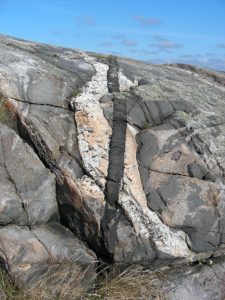
Intrusive rocks, visible on the left and right of this image, crosscut by a white pegmatite dike, in turn crosscut by a dark-colored dolerite dike. Kosterhavet National Park, Yttre Ursholmen island, Koster Islands, Sweden. Photo © Thomas Eliasson/Geological Survey of Sweden.
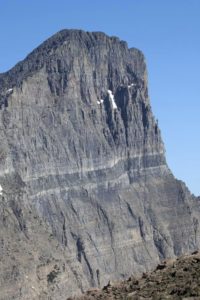
The Purcell Sill, in Montana, is well exposed on a near-vertical mountain cliff. The dark-colored sill, which exploits the layering of the surrounding Proterozoic rocks, is further highlighted by contact metamorphism related to the intrusion, which produced white metamorphic haloes around the sill. Photo © Marli Miller. Nice blog post on AGU – Mountain Beltway by Callan Bentley.
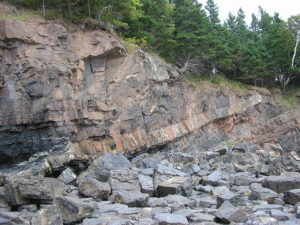
Sills are parallel to bedding and somewhat difficult to recognize at first glance, but the more prominent layer in this image consists of Middle Carboniferous igneous rocks (dolerite) that crosscut the surrounding Lower Carboniferous sedimentary rocks. Cheverie, Minas Basin South Shore, Nova Scotia, Canada. Photo © Mike Norton via wikipedia.
Why should we bother with the modes magma intrudes the crust?
Dikes and sills are extremely sensitive to the tectonic environment and the structures where they emplace and are generally much more complicated that the simple definitions given above. Sills and dikes form interconnected geometries and can exploit the geologic environment where they emplace in various way. One such example is shown in Stephens et al. (2017), where igneous sills apparently crosscut vertical layers in the surrounding rock: detailed structural analysis shows that they actually exploit pre-existing horizontal fractures.
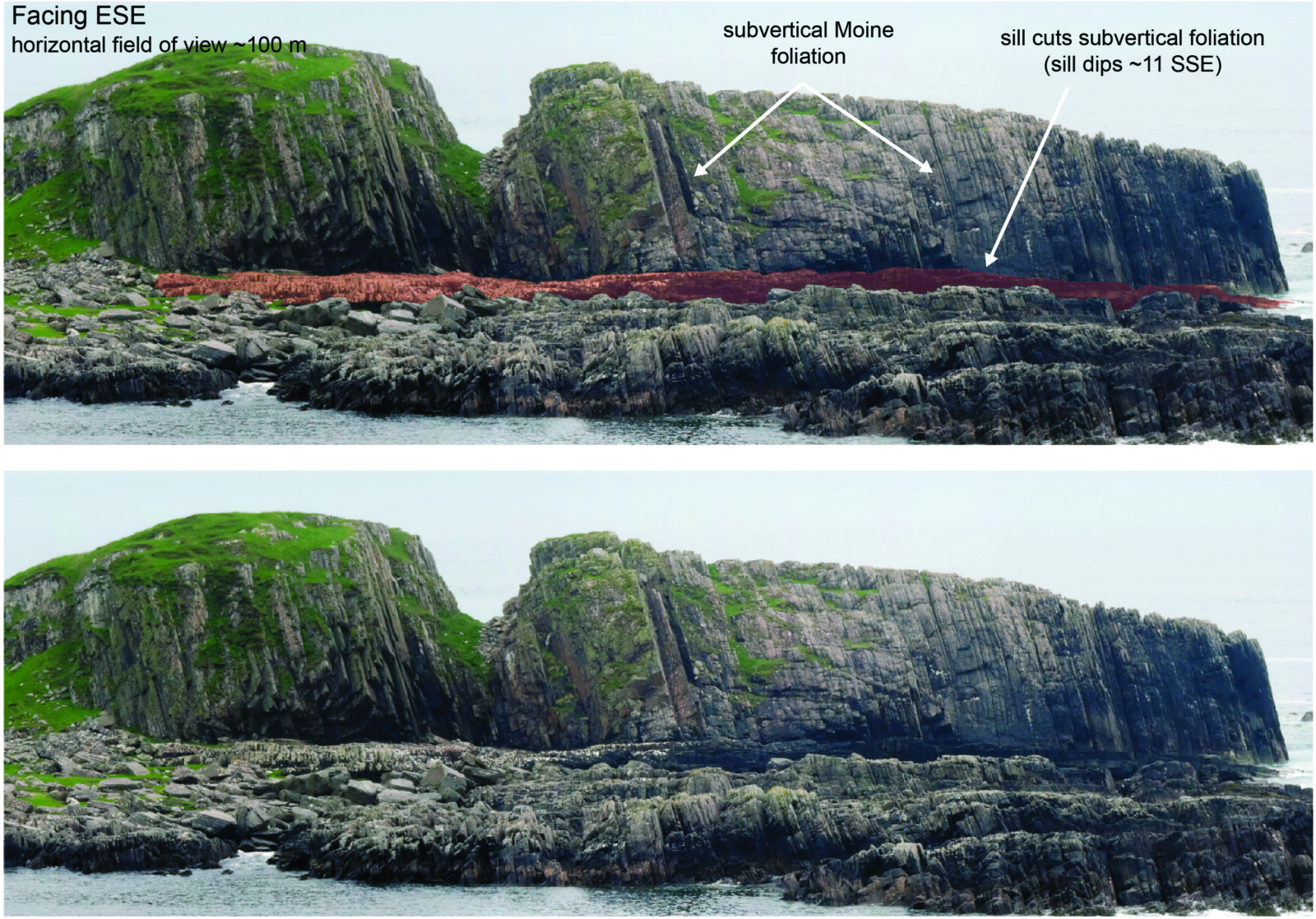
Igneous sill exploiting sub-horizontal fractures and cross-cutting the layers of the surrounding rocks. In this example, far-field tectonic forces are more important than the local structures in controlling sill geometry. Isle of Mull, Scotland. Photo © Tara Stephens via Stephens et al. (2017).
Another example of the complexity of dike-sill relationships which I personally love is this photo (below) by prof. Alan Cooper (University of Otago), which shows a sill exploiting the layering of Dalradian quartzites, linking two dikes intruding on vertical fractures (or, perhaps, these are all sills, since they all exploit foliations and fractures?)
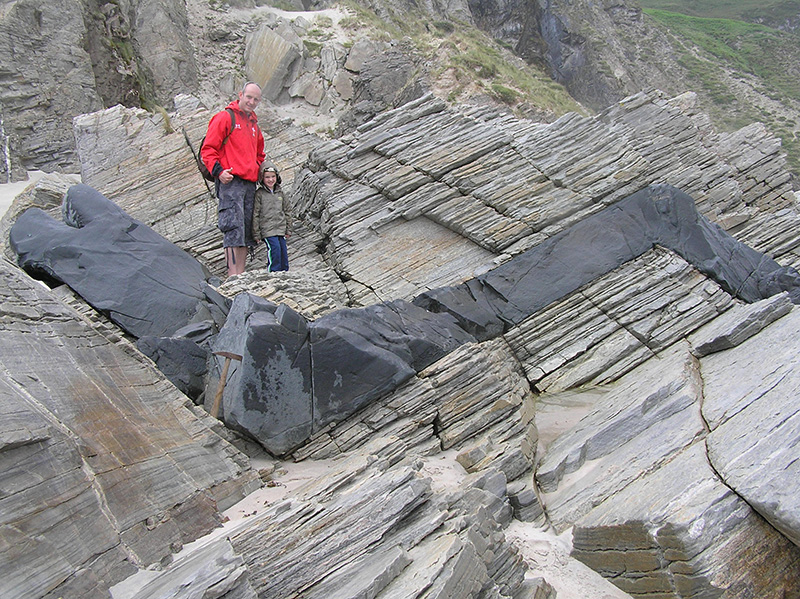
This prominent dark-colored mafic intrusion meanders through the surrounding white Dalradian quartzites, sometimes exploiting their foliation, other times their joints. Are these dykes or sills? Photo © Prof. Alan Cooper (University of Otago).
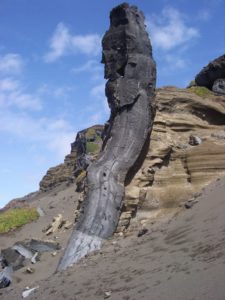
A feeder dyke in volcanic rocks on the Fayal Island (Azores, Portugal), now exposed by erosion. Feeder dykes, like this one, transport magma from below the surface to volcanic vents. Photo © Dr. Heidi Soosalu.
Why study dikes and sills?
Understanding how dikes and sills open and propagate is crucial to understand how magma is transported to volcanoes (e.g. Vigneresse, 1995; Muirjhead et al., 2012). The movement of magma in these ‘underground pipes’ is often associated with signals indicative of volcanic unrest (surface deformation, seismicity). At a larger scale, dikes, and, by extension, volcanoes, are commonly controlled by regional faults. Fissure eruptions, like that of the Cumbre Vieja mentioned earlier (Day et al., 1999), are fissure eruption, forming, indeed, at the intersection between sheet dikes and the surface of the Earth. Geophysicists and volcanologists monitor volcanoes to understand how these fissures open. Structural geologists and petrologists work together on exhumed dikes and sills to understand how magma was stored and moved within the crust (e.g. Kavanagh and Sparks, 2011; Kjøll et al., 2019). Studying dikes and sills is also important to understand past climates. Some of the largest extinction events that ever happened to our planet (like the Permian/Triassic mass extinction) coincided with enormous volcanic eruptions (Large Igneous Provinces or LIPs) and 75% of the magma emitted in these large eruptions remained in the crust in the form of dykes, sills, and other layered mafic intrusions (e.g. Svensen et al., 2007; Burgess et al., 2017). Critical resources, like Ni, Cu, Co and Platinum Group Elements occur in sulfide deposits hosted in sills (e.g. Barnes et al., 2016) and, even when these mineralizations are not hosted directly within sills, they are commonly associated with hydrothermal circulation above and driven by sills. In volcanic passive margins, sills are also associated with trapping and maturation of hydrocarbons (e.g. Lee et al., 2006).
Finally, dikes and sills are also associated with some of the largest metal ore formations on the planet, like porphyry copper deposits. This is because the pathways used by magma also control the movement of mineralizing fluids within the crust. In many cases, these fluids emanate from the crystallization of the magma itself. Understanding how these dikes form helps us investigate how these critical resources are distributed in rocks, how we can extract them, and locate other places where they can be exploited.
References and further reading
Barnes, S. J., Cruden, A. R., Arndt, N., & Saumur, B. M. (2016). The mineral system approach applied to magmatic Ni–Cu–PGE sulphide deposits. Ore geology reviews, 76, 296-316.
Burgess, S. D., Muirhead, J. D., & Bowring, S. A. (2017). Initial pulse of Siberian Traps sills as the trigger of the end-Permian mass extinction. Nature Communications, 8(1), 1-6.
Day, S. J., Carracedo, J. C., Guillou, H., & Gravestock, P. (1999). Recent structural evolution of the Cumbre Vieja volcano, La Palma, Canary Islands: volcanic rift zone reconfiguration as a precursor to volcano flank instability?. Journal of Volcanology and Geothermal Research, 94(1-4), 135-167.
Kavanagh, J. L., & Sparks, R. S. J. (2011). Insights of dyke emplacement mechanics from detailed 3D dyke thickness datasets. Journal of the Geological Society, 168(4), 965-978.
Kjøll, H. J., Galland, O., Labrousse, L., & Andersen, T. B. (2019). Emplacement mechanisms of a dyke swarm across the brittle-ductile transition and the geodynamic implications for magma-rich margins. Earth and Planetary Science Letters, 518, 223-235.
Lee, G. H., Kwon, Y. I., Yoon, C. S., Kim, H. J., & Yoo, H. S. (2006). Igneous complexes in the eastern Northern South Yellow Sea Basin and their implications for hydrocarbon systems. Marine and Petroleum Geology, 23(6), 631-645.
Menand, T. (2011). Physical controls and depth of emplacement of igneous bodies: A review. Tectonophysics, 500(1-4), 11-19.
Muirhead, J. D., Airoldi, G., Rowland, J. V., & White, J. D. (2012). Interconnected sills and inclined sheet intrusions control shallow magma transport in the Ferrar large igneous province, Antarctica. Bulletin, 124(1-2), 162-180.
Stephens, T. L., Walker, R. J., Healy, D., Bubeck, A., England, R. W., & McCaffrey, K. J. (2017). Igneous sills record far-field and near-field stress interactions during volcano construction: Isle of Mull, Scotland. Earth and Planetary Science Letters, 478, 159-174.
Svensen, H., Planke, S., Chevallier, L., Malthe-Sørenssen, A., Corfu, F., & Jamtveit, B. (2007). Hydrothermal venting of greenhouse gases triggering Early Jurassic global warming. Earth and Planetary Science Letters, 256(3-4), 554-566.
Vigneresse, J. L. (1995). Crustal regime of deformation and ascent of granitic magma. Tectonophysics, 249(3-4), 187-202.
Walker, G. P. (1993). Basaltic-volcano systems. Geological Society, London, Special Publications, 76(1), 3-38.
Acknowledgements
I warmly thank Sandy Cruden, Francesco Mazzarini, Paolo S. Garofalo, Tara Stephens, and John Faithfull for their help in writing this blog post! I also thank Alan Cooper for the permission to reuse his image. Thanks to all who contributed to my *hunt for dikes/sills* on twitter!