Volcanoes are everywhere along plate margins and on hot spots on the planet’s surface. Just in the past 2 years, we have witnessed enormous explosive eruptions such as that of the Hunga Tonga volcano which released an amount of energy equivalent to hundreds of atomic bombs, and massive lava flows, as at the Cumbre Vieja at La Palma, which was estimated to have a total volume of 80 million cubic me ...[Read More]
Features from the Field: Dikes and Sills
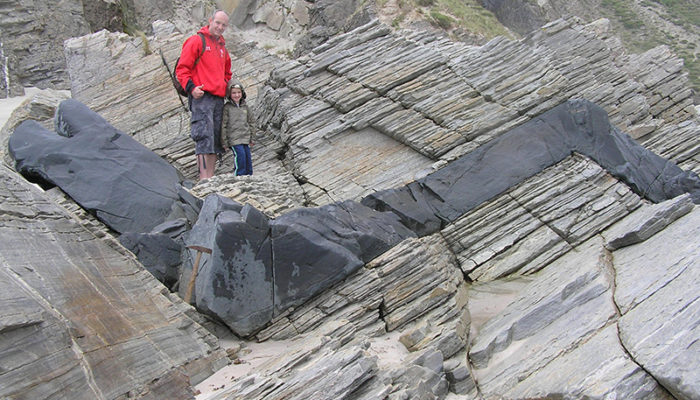