We know that climate change is affecting many aspects of what we have considered “stable” throughout a long period of modern times. One of many impacted and changing factors is snow and thereby also snow meltwater. One way to study meltwater is to trace it by the usage of stable water isotope signals and we plan to use it by asking: Are we underestimating the portions of snow meltwater in differen ...[Read More]
Did you know about the weathering crust? Five things you never knew about glacier surfaces
To the untrained eye, the melting surface of glaciers and ice sheets can look a little boring. It’s bright in some places, dark in others, and there are lots of things to fall over and (hopefully not) get your feet wet in. However, a huge range of processes are occurring both upon and just underneath the ice surface, in a 50-ish cm thick layer of ice called the weathering crust (or the “crust” for ...[Read More]
Did you know that glacier mass loss affects water resources?
The picture above shows a typical Kyrgyz summer yurt camp, located in the valley of Altyn-Arashan, Kyrgyzstan. The stream you see flowing through comes from the glacier-fed lake of Ala-Kul, the gorgeous turquoise water featured below. The families who live there during summer have done so for generations, and travel up with their herds of horses and cows. The stream provides the water they need fo ...[Read More]
Running a live stream of proglacial processes
In Switzerland, nothing is really remote, but some places are more so than others. Dense infrastructure networks typically provide convenient access to research sites in the Alps where it is difficult to feel far away from home. However, this is not always the case… For us, our home for the summer is a bit different. We work at 2400 m above sea level in Southern Switzerland, in a narrow vall ...[Read More]
Exploring the hidden plumbing of glaciers with Cryoegg
Plumbing is something we take for granted: the pipes that bring us water to wash and drink, and the pipes that take the waste water away again. We see the taps and basins in our kitchen and bathroom – but the pipes are hidden away under the floor or inside the walls – and we mostly ignore them until there’s a leak or a blockage! It turns out that glaciers have plumbing too – and ...[Read More]
Image of the Week – Drilling into a Himalayan glacier
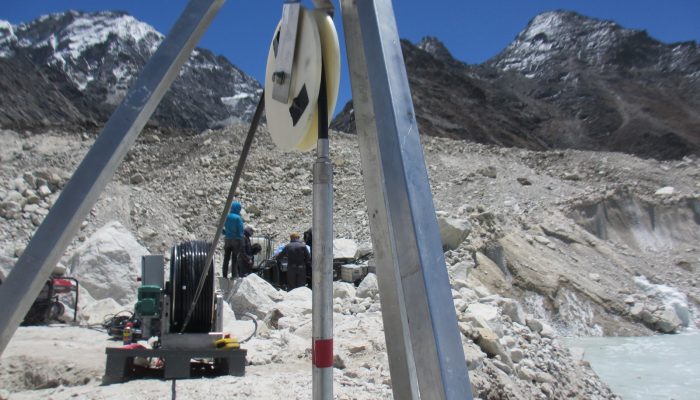
How water travels through and beneath the interior of debris-covered glaciers is poorly understood, partly because it can be difficult to access these glaciers at all, never mind explore their interiors. In this Image of the Week, find out how these aspects can be investigated by drilling holes all the way through the ice… Hydrological features of debris-covered glaciers Debris-covered glaciers ca ...[Read More]
Image of The Week – A Game of Drones (Part 1: A Debris-Covered Glacier)
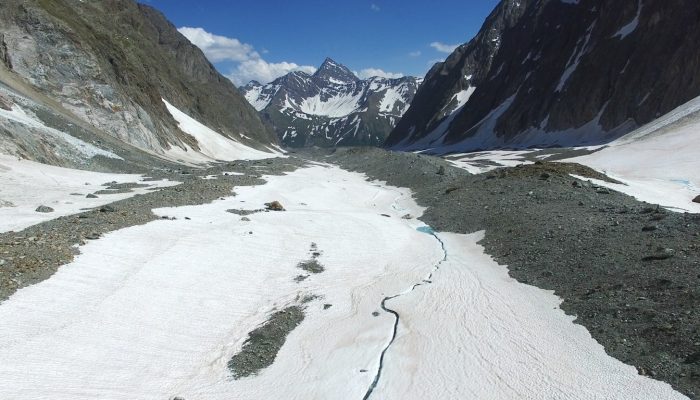
What are debris-covered glaciers? Many alpine glaciers are covered with a layer of surface debris (rock and sediment), which is sourced primarily from glacier headwalls and valley flanks. So-called ‘debris-covered glaciers’ are found in most glacierized regions, with concentrations in the European Alps, the Caucasus, Hindu-Kush-Himalaya, Karakoram and Tien Shan, the Andes, and Alaska and the weste ...[Read More]