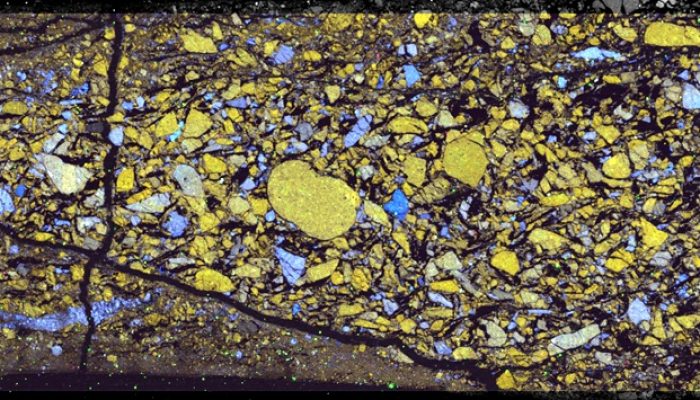
André Niemeijer, Assistant Professor, Department of Earth Sciences at Utrecht University, the Netherlands
In this blogpost we will go on a tour of the High Pressure and Temperature (HPT) Laboratory at Utrecht University and learn about some of the interesting science done there.
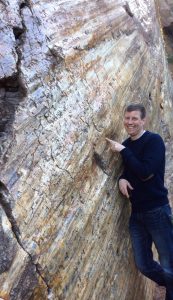
André Niemeijer next to a striated fault surface. Credit: André Niemeijer.
André’s main interest is fault friction and all the various processes that are involved in the seismic cycle. This includes the evolution of fault strength over long and short timescales, the evolution of fault permeability and the effects of fluids. His current research is aimed at understanding earthquake nucleation and propagation by obtaining a better understanding of the microphysical processes that control friction of fault rocks under in-situ conditions of pressure, temperature and fluid pressure.
Most of the deformation in the Earth’s brittle crust occurs on and along faults. Fault movement produces fine-grained wear material or gouge, which is very prone to fluid-rock interactions and mineral reactions (Wintsch, 1995). It has long been recognized that the presence of a fluid allows for deformation to occur at much lower differential stresses than without.
Pressure solution
One of the mechanisms by which this deformation occurs is pressure solution (alternatively termed “solution-transfer creep” or “dissolution-precipitation creep”). This mechanism operates through the dissolution of materials at sites of elevated stress, diffusion along grain boundaries and re-precipitation at low stress sites (e.g. pores). Pressure solution is an important diagenetic process in sandstones and carbonates as evidenced by the presence of stylolites in many carbonate rocks, which are often used as counter tops and floors (particularly in banks, I noticed). In addition, it has been suggested that pressure solution plays an important role in the accommodation of (slow) shear deformation of faults (Rutter & Mainprice, 1979) and possibly in controlling the recurrence interval of earthquakes (Angevine, 1982).
Fluid-rock interactions in the lab
Experimentally, it is challenging to activate pressure solution or mineral reactions in the laboratory, because they are typically slow processes. Moreover, it is difficult to find evidence of their operation. We have used a unique hydrothermal rotary shear apparatus, which is capable of temperatures up to 700 °C to activate pressure solution in fine-grained quartz gouges. We were able to prove that new material was precipitated by using a combination of state-of-art electron microscopy techniques that involve cathodoluminescence (CL).
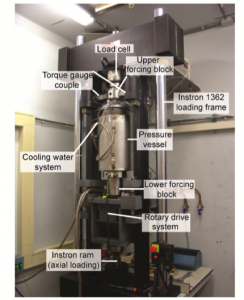
The hydrothermal rotary shear apparatus at the HPT laboratory at Utrecht University, the Netherlands. Credit: André Niemeijer.
Signature of pressure solution
The CL signal of a mineral depends on the type and level of impurities and defects that are present. We used quartz derived from a single crystal which showed relatively uniform CL. Because our apparatus has various metal alloy parts, small amounts of aluminium are present in the fluid. Aluminium can be incorporated in newly precipitated quartz, which gives a different CL signal. This allows us to map the locations where quartz has newly formed and link this to the experimental data. Taken together, we can use these to derive and constrain microphysical models for fault slip that can be used to extrapolate to natural conditions (e.g. Chen & Spiers 2016, van den Ende et al., 2018).
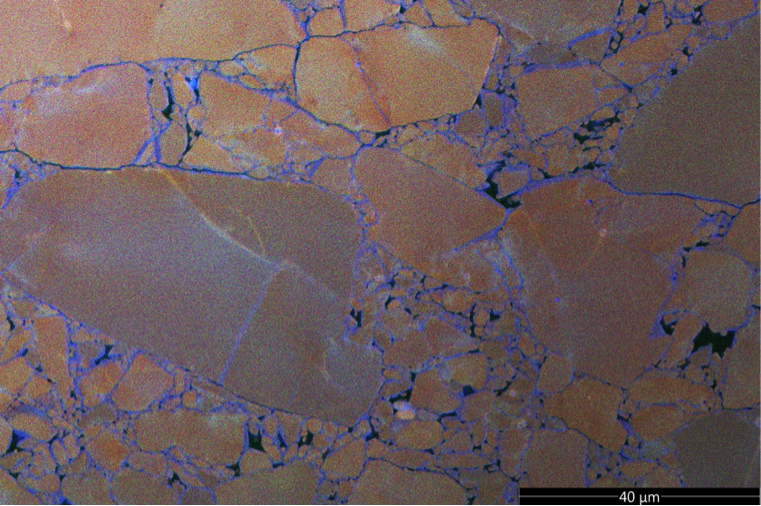
RGB overlay of secondary electron and cathodoluminescence signals in a deformed quartz sample. Newly precipitated quartz shows up in a blue colour. Credit: Maartje Hamers.
Mineral reactions
Outcrops of natural faults often show evidence for enhanced mineral reactions with increasing shear strain. For instance, the Zuccale fault (Isle of Elba, Italy) has a high content of talc in the highest strained portion of the fault (Collettini & Holdsworth, 2004). Talc is a frictionally weak mineral and its presence in the Zuccale fault provides an explanation for the possibility of slip along this low-angle normal fault. We were able to produce talc experimentally from mixtures of dolomite and quartz in only 3-5 days of shearing at low velocity. This shearing was accompanied by major weakening, with friction dropping from 0.8 to as low as 0.3. The reaction to talc is sensitive to temperature and fluid composition. At slightly higher temperature, we produced diopside and forsterite which are frictionally unstable and generated audible laboratory earthquakes.
Identifying reaction products
We tried a whole range of different analytical techniques to identify the reaction products. Despite the obvious frictional weakening that we observed, talc was only observed in two samples with x-ray diffraction (XRD). Fourier-transform Infrared analysis, on the other hand, proved to be very sensitive to talc and has the big advantage that only a small amount of material is needed (~70 mg). Electron microscopy with EDS-analysis (Energy Dispersive X-ray Spectroscopy) proved helpful to some extent, because it shows the phase distribution. However, the small size of reaction products gives a mixed chemistry, which complicates the identification of reaction products. Finally, to positively identify the various phases in the different samples, we employed Raman mapping.
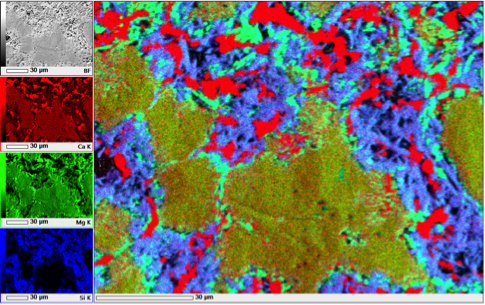
RGB overlays of EDS analyses of samples deformed at 300 °C (left) and 500 °C (right). Dolomite appears in yellow, quartz in blue, calcite in red, talc in cyan in the left image, while dolomite is orange, calcite is red, diopside is purple and forsterite is cyan in the right image. Credit: André Niemeijer.
Outlook
Our studies have shown that reactions can be quite rapid in fine-grained fault gouges. These reactions can have a profound effect on both fault strength and stability but are typically ignored in large-scale models of the seismic cycle. Incorporating reactions requires models that can account for the effect of stress and grain size reduction on the development of faults, which is not an easy task, but is a necessary ingredient to understand the long-term behavior of faults.
Edited by Derya Gürer
References
- Angevine, C. L., Turcotte DL, Furnish MD. (1982) Pressure solution lithification as a mechanism for the stick-slip behavior of faults. Tectonics 1 (2), 151-160 doi:10.1029/TC001i002p00151.
- Chen, J. and Spiers CJ. (2016) Rate and state frictional and healing behavior of carbonate fault gouge explained using microphysical model. Journal of Geophysical Research: Solid Earth 121 (12), 8642-8665 doi:10.1002/2016JB013470.
- Collettini, C. and Holdsworth RE. (2004) Fault zone weakening and character of slip along low-angle normal faults: Insights from the Zuccale fault, Elba, Italy. Journal of the Geological Society 161 (6), 1039-1051 doi:10.1144/0016-764903-179.
- E H Rutter, D H Mainprice (1979)On the possibility of slow fault slip controlled by a diffusive mass transfer process. Gerlands Beitr. Geophysik, Leipzig 88 (1979) 2, S. 154-162.
- van den Ende, M. P. A., Chen J, Ampuero J., Niemeijer AR. (2018) A comparison between rate-and-state friction and microphysical models, based on numerical simulations of fault slip. Tectonophysics 733, 273-295 doi:10.1016/j.tecto.2017.11.040.
- Wintsch, R. P., Christoffersen R, Kronenberg AK. (1995) Fluid-rock reaction weakening of fault zones. Journal of Geophysical Research: Solid Earth 100 (B7), 13021-13032 doi:10.1029/94JB02622.