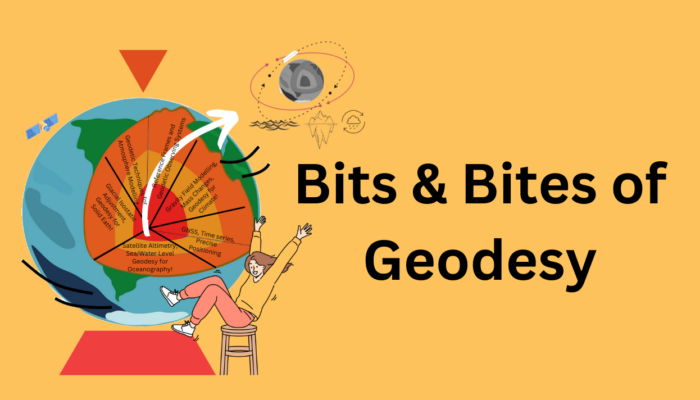
In a previous post, Thomas Frederikse briefly explained how geodesy is used to observe sea level change. In this part of Bits & Bites, Bene delves deeper into the topic.
Rising sea levels are one of the consequences of climate change that already impacts people in low-lying coastal regions, and the future prospects are not exactly encouraging as well. We know that rising sea levels are caused by warmer water that expands in volume and by melting ice caps and glaciers that add mass to the oceans. On the global average, sea levels were rising at a rate of 3.7 mm/year between 2006 and 2018 (IPCC report 2023), and this rate is accelerating. This is not only disturbing but a very essential piece of information about what the future holds for us.
Observing sea level with tide gauges
But how do we know the rate of sea level rise so precisely? Traditionally, sea level is observed with tide gauges (imagine you fix a ruler to a wall and record the readings of water level). There are great projects to collect and harmonise all the tide gauge data in the world such as the PSMSL (Permanent Service for Mean Sea Level), but two main problems remain:
- First, tide gauges can per nature only be installed at coasts and are often found in harbours, resulting in a very uneven spatial coverage with a bias to the northern hemisphere and to countries with larger financial means.
- Second, the ground where the tide gauge is fixed is not stable. As an example, extracting a lot of groundwater causes the material above the reservoir to subside. In some places, subsidence of the ground is an even bigger problem than sea level rise, for example in Indonesia.
Consequently, what a tide gauge observes is the combination of absolute sea level changes and vertical land motion. There are means to remove the effect of vertical land motion from the tide gauge observations, for example by using the vertical component of measurements from permanent GNSS stations (GPS is the American GNSS system) or by employing information from GIA models.
Satellite radar altimetry
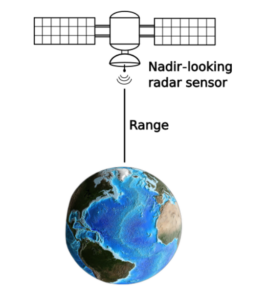
Fig. 1: With satellite altimeters, we can measure the distance, also called range, between satellite and the Earth’s surface. The altitude of the satellite is known, and subtracting the range leads to an estimate of the sea surface height.
Luckily, geodesists are very good at inventing fancy satellites! With satellite altimetry, we don’t only get data with high global coverage but also the absolute sea level changes relative to a reference surface based on the knowledge of what and where the centre of the Earth is (Where is the centre of the Earth? Why do we care?). The principle is to measure the distance between the satellite and the sea surface. This distance is also called “range” (Fig. 1). As the position of the satellite in orbit is known, for example from GNSS and DORIS observations, we can compute the absolute sea surface height from the difference between the satellite altitude and the range.
To put it simply, satellite radar altimeters are active sensors that use microwave radiation to work. It’s like having a microwave on a satellite! Although altimeters operate mostly in the Ku-, Ka- or C-band with frequencies between 5 and 35 GHz, whereas your kitchen microwave works with around 2.5 GHz. The radar pulse is directed vertically downwards (also called nadir direction), and therefore one observation covers only one point with a footprint of about 2 km – 30 km in diameter, depending on the technique and the roughness of the sea surface. That’s why the new SWOT (Surface Water and Ocean Topography) mission launched in December 2022 is expected to revolutionise our knowledge of the Earth’s surface waters. By combining the signals from two simultaneously measuring SAR (Synthetic Aperture Radar) sensors, SWOT observes entire swaths with a higher resolution than was possible with classical altimetry.
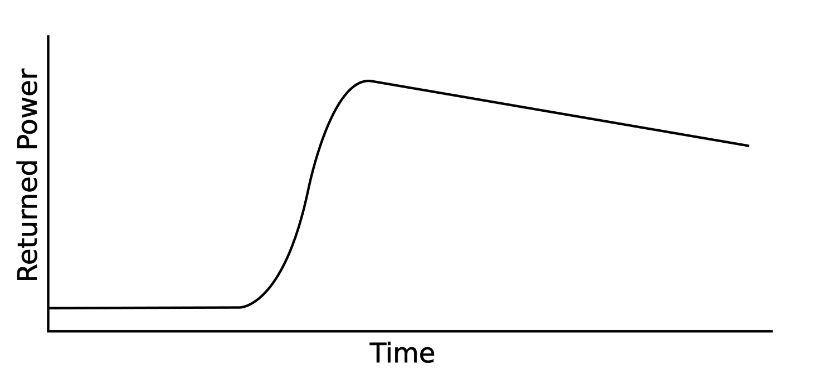
Fig. 2: Over the open ocean, waveforms (the returned energy over time) often follow a typical shape that can be parametrised in the Brown model.
Now that we know how altimeters work, let’s talk about what a radar pulse can say about the range between the satellite and the sea surface. In principle, we can measure the pulse’s two-way travel time and multiply it by the speed of light to get the distance. However, the sensor does not only measure the time difference between outgoing and incoming pulse but records the entire reflected signal. The curve of this reflected signal over time is called a waveform. Over the open ocean, this waveform has a predictable shape which can be approximated by a function called the “Brown model” (Fig. 2). By fitting this model to an individual waveform, we can estimate parameters related to the sea level, to the significant wave height, and even to the wind speed. The process of fitting a model to a waveform is called “retracking”, and the algorithm that performs this task is called a “retracker”. There are different retrackers specialised in different types of locations. Especially over coastal areas, lakes, or rivers, the waveforms do not adhere to the Brown model found in waveforms over the open ocean. Due to the influence of land in the altimeter footprint, waveforms can adopt very different shapes (Fig. 3) that require specialised retrackers.
Fig.3: As soon as land enters the footprint of the radar pulse, the waveforms differ from the Brown model, for example by exhibiting several peaks (image courtesy https://sentinel.esa.int).
In conclusion, there is no single technique that provides sea levels with high temporal resolution and global coverage. Over the last three decades, altimetry became a valuable source of data about sea level changes, that complements observations from tide gauges. In the end, the choice between using tide gauge data or satellite altimetry depends on the application and the data availability at the study site.
References Vignudelli, Stefano, Andrey G. Kostianoy, Paolo Cipollini, and Jérôme Benveniste, eds. Coastal Altimetry. Berlin, Heidelberg: Springer Berlin Heidelberg, 2011. https://doi.org/10.1007/978-3-642-12796-0. Abdalla, Saleh, Abdolnabi Abdeh Kolahchi, Michaël Ablain, Susheel Adusumilli, Suchandra Aich Bhowmick, Eva Alou-Font, Laiba Amarouche, et al. “Altimetry for the Future: Building on 25 Years of Progress.” Advances in Space Research, 25 Years of Progress in Radar Altimetry, 68, no. 2 (July 15, 2021): 319–63. https://doi.org/10.1016/j.asr.2021.01.022.