It is a pleasure for the EGU Cryoblog team to present a new post category: Cryo-institutes around the world! There are many institutes working on cryosphere-related research spread around the world. The aim of this new category is to highlight the cool research that is carried out at these institutes, showing off our multi-faceted cryo-related science. In this opening post, Ashley Morris will pres ...[Read More]
The Norwegian Polar Institute
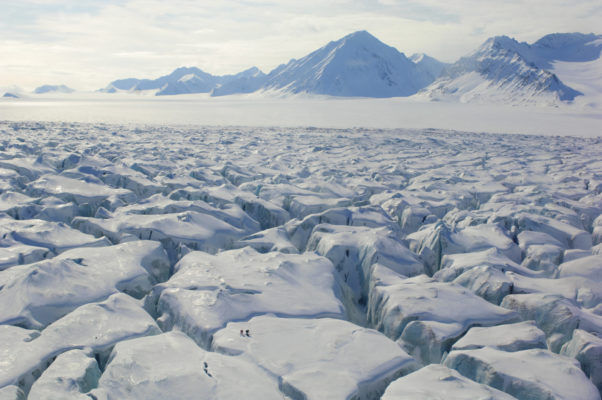