Last week, the EGU Cryosphere Blog (“Cryoblog” for the regulars) team was contacted by a reader who stumbled upon very curious ice formations while taking a morning walk in rural Berkshire, England. This was right after a few nights with below-freezing temperatures and snowfall. He asked us whether we could explain to him what these ice mushrooms were and how they formed. Very curious and always k ...[Read More]
Lost in transl[ice]tion…
![Lost in transl[ice]tion…](https://blogs.egu.eu/divisions/cr/files/2020/10/MainFigure-700x292.png)
Three years have passed since sea-ice scientists from both climate modeling and remote sensing backgrounds met for an international workshop in Hamburg. The goal was to discuss how to further improve our understanding of sea ice and reduce uncertainties in climate models and observations (see this previous post). One suggestion was to work on observation operators. Let’s see what has happened in t ...[Read More]
Did you know… the difference between sea-ice area and sea-ice extent?
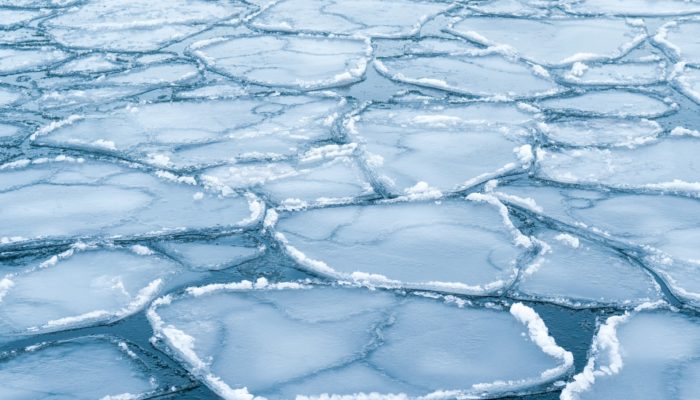
At the beginning of March, just over a month ago, sea ice in the Arctic reached its annual maximum extent. As currently all media attention is focused on other news, you might have missed that, once again, this maximum fell below the 1981 to 2010 average maximum extent. When reading headlines about such sea-ice facts, you may have been confused by the seemingly interchangeable use of “sea-ice exte ...[Read More]
Did you know about… nature’s street lights for Santa Claus?
Only a couple of days left until Santa Claus’ big night! Once again the beardy guy in the red and white suit will fly around the world with his sledge pulled by his strong reindeers to make young and old children happy! But how does he navigate in the dark? Luckily, nature provides some solutions, for example light pillars… Light pillars form when ice particles are suspended in the air. These part ...[Read More]
Vamos a la playa!
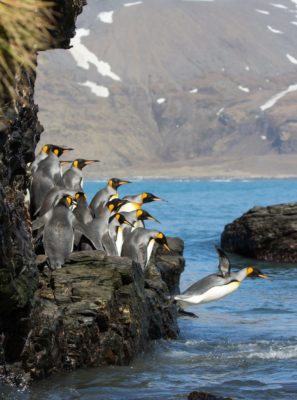
A new heatwave is currently hitting Europe. In this context, the icy landscapes of the Arctic, Antarctica or the high mountains appear to be the perfect destination to cool down! As this is not necessarily an option for everyone, we alternatively recommend to find the next river, lake, or ocean and jump in, just like the penguins in the picture above! This heatwave is also a clear sign that August ...[Read More]
Image of the Week – Icy expedition in the Far North
Many polar scientists who have traveled to Svalbard have heard several times how most of the stuff there is the “northernmost” stuff, e.g. the northernmost university, the northernmost brewery, etc. Despite hosting the four northernmost cities and towns, Svalbard is however accessible easily by “usual-sized” planes at least once per day from Oslo and Tromsø. This is not the case for th ...[Read More]
Image of the Week – Edible cryosphere at EGU 2018!
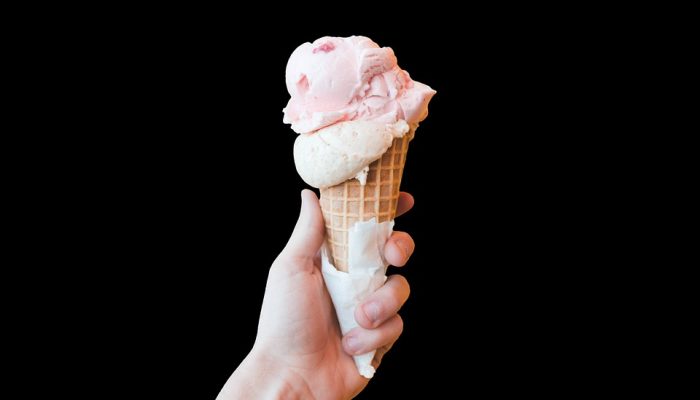
It is time again for the annual family meeting of the European Geosciences Union! A lot of interesting talks, posters and events are waiting for you! But this also means you will have to use your brain a lot to concentrate and understand what is going on… Every year, around 15,000 geoscientists meet in Vienna for the EGU General Assembly (see our guide to navigating EGU 2018). It is an exciting ev ...[Read More]
Image of the Week – Fifty shades of snow
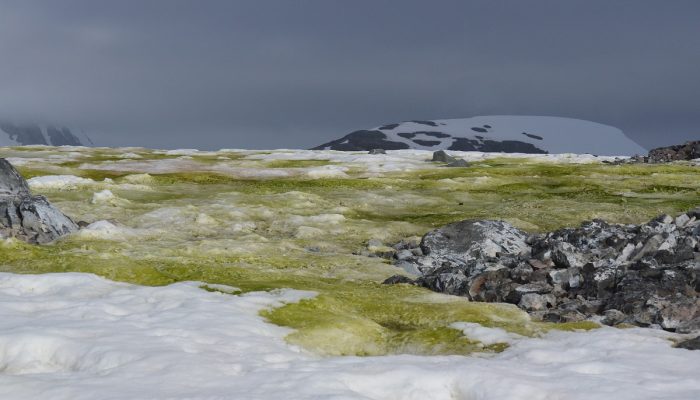
When I think of snow, I tend to either think about the bright white ski slopes in the mountains or the large white areas in the Arctic. However, natural phenomena can lead to colorful snow. Our Image of the Week shows snow can be green! Snow can also turn orange, pinkish, grey and even yellow… But where do these different shades of snow come from? White The most common color of snow is white (see ...[Read More]
Image of the Week – The ups and downs of sea ice!
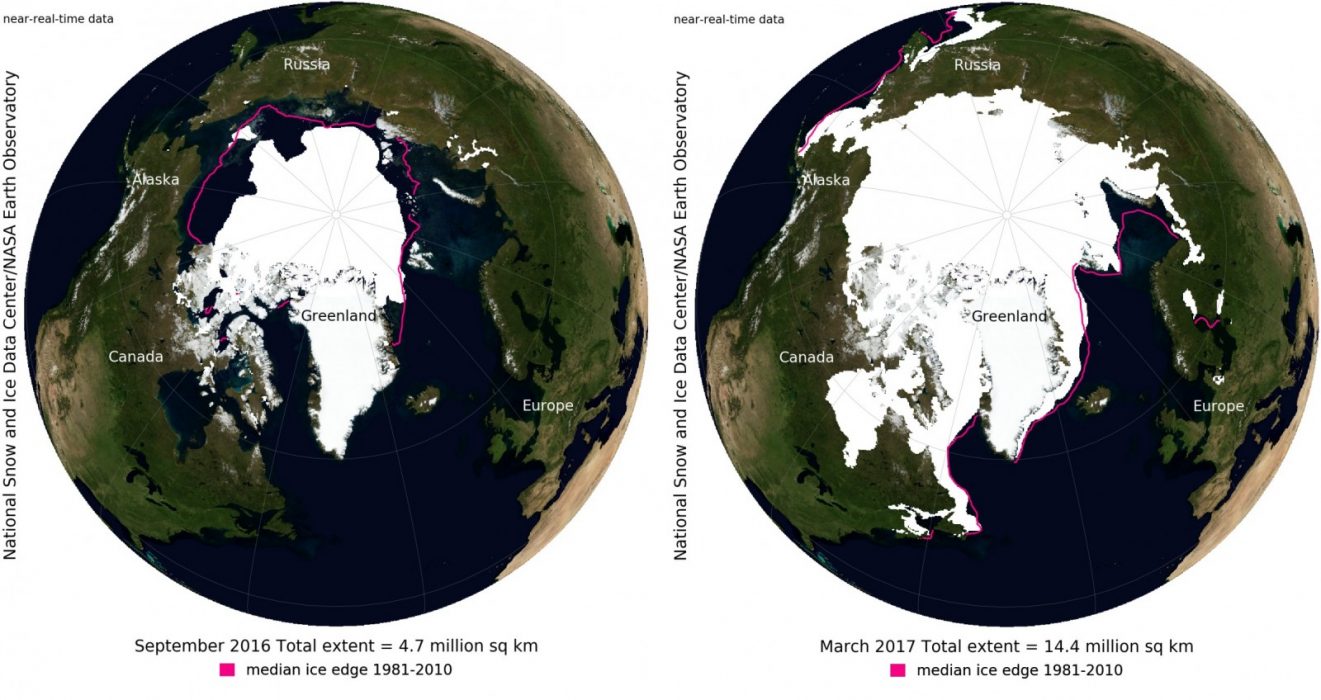
The reduction in Arctic sea-ice cover has been in the news a lot recently (e.g. here) – as record lows have been observed again and again within the last decade. However, it is also a topic which causes a lot of confusion as so many factors come into play. With this Image of the Week we will give you a brief overview of the ups and downs of sea ice! In general, Arctic sea ice is at its minim ...[Read More]
Image of the Week – Let it snow, let it snow, let it snow…
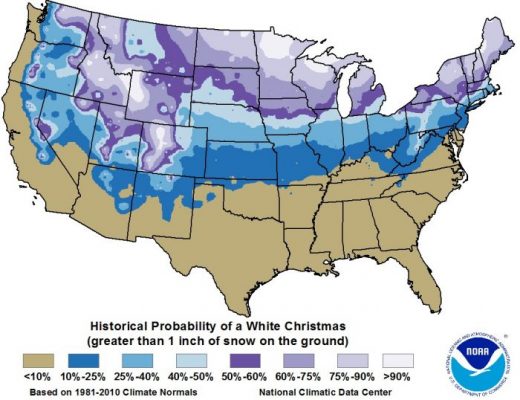
Christmas is coming to town and in the Northern Hemisphere many of us are still dreaming of a white Christmas, “just like the ones we used to know”. But how likely is it that our dreams will come true? What is the definition of a White Christmas ? Usually Christmas can be defined as a “White Christmas” if the ground is covered by snow on either Christmas Eve or Christmas Day depending on local tra ...[Read More]