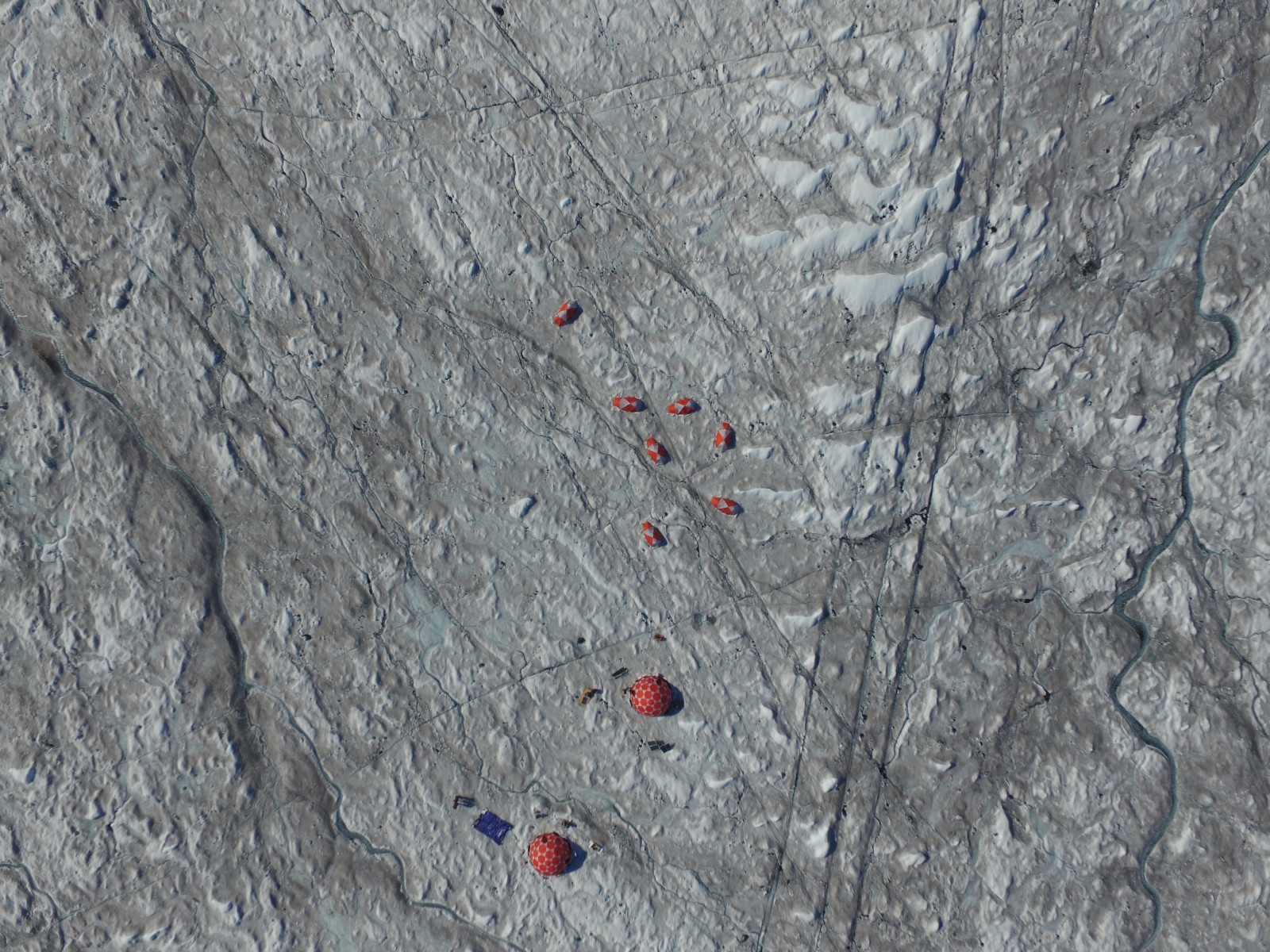
Most of the energy that drives glacier melting comes directly from sunlight, with the amount of melting critically dependent on the amount of solar energy absorbed compared to that reflected back into the atmosphere. The amount of solar energy that is reflected by a surface without being absorbed is called the albedo. A low albedo surface absorbs more of the energy that hits it compared to a high albedo surface. Our Image of the Week shows patches of dark grey-brown algal blooms on the Greenland Ice Sheet, giving the surface a surprisingly low albedo.
The colour of ice
Clean ice and snow are among the most reflective natural materials on Earth’s surface making them important ‘coolers’ in Earth’s climate system. The term ‘albedo’ describes how effectively a material absorbs or reflects incoming solar energy – it is the ratio of downwelling light arriving at a surface to the amount of upwelling light leaving it. The albedo of fresh, clean snow can be as high as 90%, meaning that out of all the solar energy reaching the surface only 10% is absorbed. However, the albedo of ice and snow can vary widely. This is important because the albedo determines how much of the incoming solar energy is retained within the snow or ice and used to raise the temperature or drive melting. It therefore controls snow and ice energy balance to a large extent.
There are several reasons why the albedo of snow and ice can vary. First, once ice crystals begin to melt they lose their delicate structures that efficiently scatter light and develop rounded granular shapes. Meltwater generated by snow or ice melt fills the gaps between the grains, promoting forward scattering of light deeper into the ice, rather than scattering back towards the surface. This increases the distance travelled through media where absorption can occur, and therefore lowers the albedo as the light is less likely to escape the material after it enters. The more melt, the greater this effect. Second, other materials such as dust or rock debris can enter the snow or ice. These ‘impurities’ generally absorb light more effectively than the ice crystals themselves and therefore reduce the albedo. However, this depends upon their concentration, optical properties and proximity to the surface. Additionally, whether the impurities are inside or outside the ice crystals, where on the planet the material is and the time of day are also important.
Any impurity that darkens a mass of ice or snow increases the amount of solar energy absorbed compared to when the material is impurity-free. This means that impurities promote melting, which is in itself an albedo reducing process. Therefore, the impact of impurities on albedo is non-linear and greater than the direct effect of their absorption alone. There are many different impurities that commonly lower the albedo of ice and snow, including mineral dusts and black carbon (e.g. from fossil fuel combustion). However, there is also a growing literature on another form of impurity that darkens ice and snow on glaciers and ice sheets on both hemispheres: biological growth (also see this previous post). Algae are the primary biological albedo-reducers on ice and snow. Photosynthetic microalgae bloom on the surface where light is abundant, which provides them with energy that they use to turn carbon dioxide and water into sugars. This in turn provides food for other microorganisms. In doing so, they darken the ice surface simply because the algal cells are more effective absorbers than the ice crystals. However, as the algae become exposed to increasing light intensities, they produce pigments that act as sun shields, protecting their cellular machinery from the damaging effects of too much light. This effect enhances the biological darkening and increases the energy absorbed within the snow or ice.
Biological darkening
There are several distinct microbial habitats on glaciers and ice sheets. Snow algae are a feature of melting snowpacks that colour snow surfaces green early in the year and red later because prolonged exposure to sunlight causes them to produce red ‘sunscreen’ pigments (see this previous post). Their influence on snow albedo has yet to be determined, although they have been shown to change the amount of visible light reflected from the surface (Lutz et al., 2014) and in Antarctica they have been shown to influence light absorption at depth within the snowpack (Hodson et al., 2017). Some bacteria have been identified feeding upon the algae, and the algal blooms also provide food for red coloured ice worms. This is probably why, in ‘The History of Animals’, Aristotle wrongly attributed the red discoloration of patches of snow to red worms rather than pigmented algae!
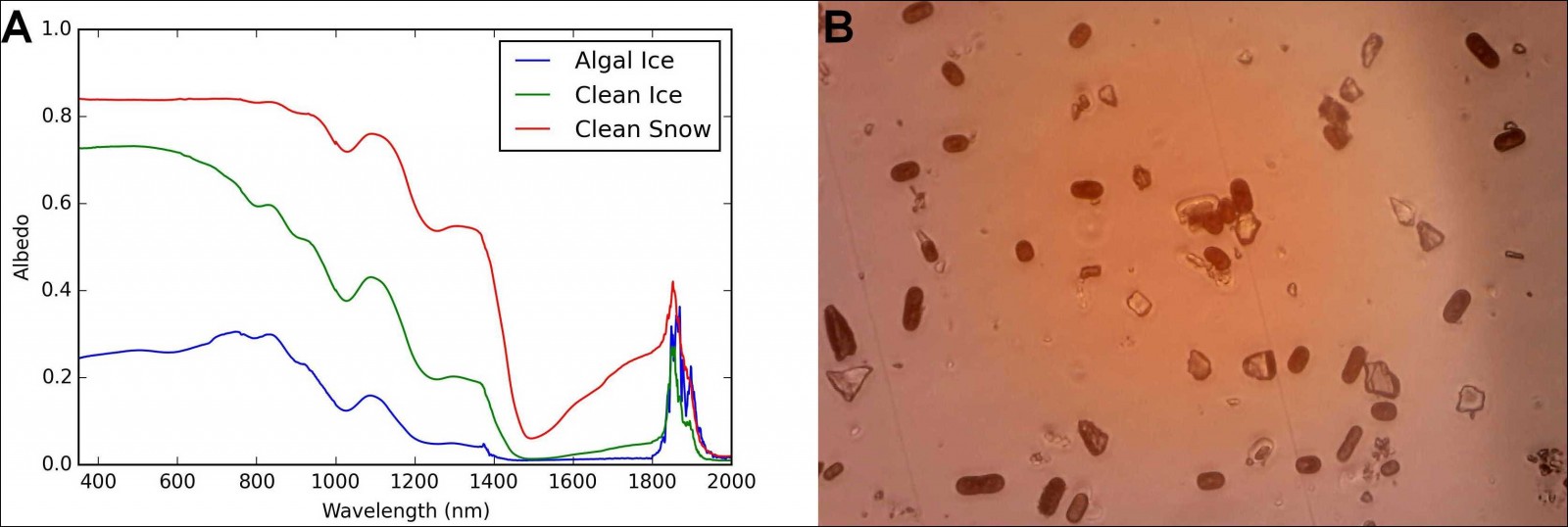
Fig. 2: (a) Albedo for clean snow, bare ice and ice with an algal bloom measured on the Greenland Ice Sheet in July 2017. (b) Microscope image of melted surface ice from the Greenland ice sheet. The red oval shaped particles are ice algae and the angular, clear particles are mineral dust fragments. [Credit: A: J. Cook, B: C. Williamson]
On ice, a different species of algae exists in a thin liquid water film on the upper surface of melting ice crystals. These algae are also photosynthetic but are not bright green or red, but rather grey, brown or purple. They produce a purple pigment that acts as a UV shield that protects their delicate intracellular machinery from excessive light energy. The side effect of this is that the algae become very dark and have an albedo-lowering effect on the ice surface (see our Image of the Week). Ice with algae has a lower albedo than clean ice (Fig 2a) but, up to now, the magnitude of the biological darkening effect has not been quantified because of difficulties isolating algal darkening from that of mineral dusts, soot and the changing optical properties of the ice itself. This also limits our capability to map these algae using remote sensing. Samples of dark coloured ice examined under the microscope clearly show the presence of an algal community darkening the ice (Fig 2b).
In addition to surface-dwelling ice algae, microbial life exists in small pits known as cryoconite holes (see also this previous post). At the bottom of these holes exists a thin layer of granules comprising living microbial cells, dead cells, biogenic molecules, mineral fragments and soot. The organic matter in these granules is very dark, so they warm up when illuminated by the sun and melt into the ice. The relationship between cryoconite and ice surface albedo is complex because, although the cryoconite is dark, the hole geometry hides the granules beneath the ice surface.
Implications for the future of glaciers and ice sheets
The challenge facing scientists now is to quantify the bioalbedo effect by determining the optical properties of individual algal cells and remotely assessing their spatial coverage at the scale of entire glaciers and ice sheets. This will require new methods to be developed for detecting living cells from the air or space. Then, we must understand the factors controlling their growth, so we can predict biological darkening of ice in future climate scenarios. It is possible that algal coverage will increase as glaciers and ice sheets waste away because algae bloom where there is liquid melt water. Because of the darkening effect, an increasingly widespread algal ecosystem in a warming climate will accelerate the demise of its own habitat by enhancing glacier and ice sheet retreat.
Further reading
- Lutz, S, et al., (2014): Variations of algal communities cause darkening of a Greenland glacier. FEMS Microbiology Ecology, 89(2). 402-414.
- Hodson, A., et al., (2017): Microbes influence the biogeochemical and optical properties of maritime Antarctic snow. Journal of Geophysical Research: Biogeosciences, 122(6): 1456-1470
- Eli Kintisch (2017): The Great Greenland Meltdown, Science Magazine.
- Black Carbon: the dark side of warming in the Arctic
- Image of the Week – Micro-organisms on Ice!
- Image of the Week – Fifty shades of snow
- Black and Bloom: blackandbloom.org
- Dark Snow Project: darksnow.org
Edited by Scott Watson and Clara Burgard
Joseph Cook is a Postdoctoral Research Associate on NERC’s Black and Bloom project based at the University of Sheffield, UK where his remit is the measurement and modelling of surface albedo on the Greenland Ice Sheet. His background is in biotic-abiotic interactions on ice. He tweets as @tothepoles and blogs at http://tothepoles.wordpress.com. Contact Email: joe.cook@sheffield.ac.uk