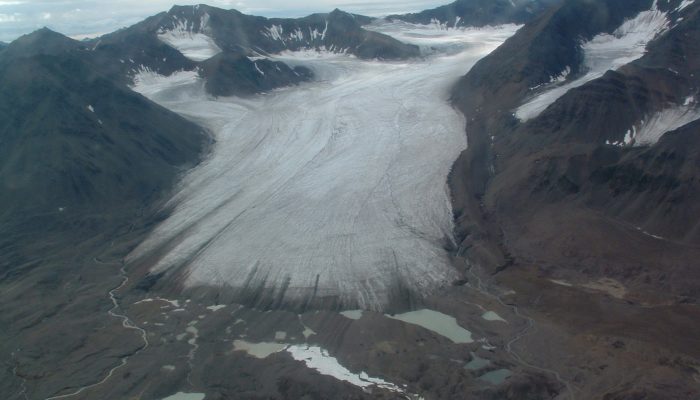
Glacier erosion happens at the interface between ice and the ground beneath. Rocks are ground down to dust and landscapes shaped by the flowing ice. While these might be hotspots for erosion, the dark and nutrient-poor sites are unlikely environments for biological activity. However, experiments suggest there may be novel sources of energy powering subglacial microbial life…
Where there is water, there is life…
Glaciers, ice sheets and ice caps cover around 11% of the earth’s land surface. At least 50% of the beds of these ice masses have temperatures at melting point due to the high pressure beneath the weight of the ice masses (Oswald and Gogineni, 2012). Liquid water is therefore present at the ice-bedrock interface in these areas. Additionally, erosion is a frequent feature of larger ice masses, and involves crushing and fracturing of bedrock. Consequently, recently crushed and wetted rock is a common feature of glacier and ice sheet beds. The adage that “where there is water, there is life” holds true for all glacier beds sampled to date, and for the only subglacial lake directly sampled beneath Antarctica, Subglacial Lake Whillans. Still, there is a large spectrum of different aquatic subglacial habitats beneath glaciers and ice sheets. The subglacial environments host genetically and functionally diverse microbial ecosystems capable of accelerating rock weathering (Montross et al., 2013), influencing global carbon cycles (Wadham et al., 2012) and productivity in adjacent oceans (Death et al., 2014).
How long can life survive beneath large ice masses?
However, the maintenance and longevity of these ecosystems is currently an area of uncertainty. Subglacial debris contains chemicals such as sulphides and organic matter that provide energy to sustain subglacial life (Hamilton et al., 2013). This is particularly important in areas close to the margin where melting water from the surface enters through moulins and crevasses and transports O2 and other biologically useful compounds such as DOC (dissolved organic carbon), POC (particulate organic carbon) and nutrients (such as Nitrogen and Phosphorus) to the bed. However, in the interior zones of ice sheets, the direct input of these species to the bed is negligible because hydrological connections between surface and bed do not exist. The O2 that is added to the bed in these locations is limited to gas bubbles in the basal ice which is geothermally melted or melts as regelation waters form and refreeze as ice flows around irregularities of the bedrock . This is problematic for the longer term maintenance of life in subglacial lakes and other aquatic environments beneath the ice sheet interiors, such as swamps and ice stream beds, because there is a lack of dissolved oxygen, and there is little energy derived from oxidants interacting with organic matter and sulphides. Further, the supply of potentially reactive organic matter, for example within former marine sediments, is finite and decreases over time, Therefore, subglacial life beneath ice sheet interiors is destined to expire unless new and sustainable sources of energy can be generated at the bed.
Hydrogen seems to be the miraculous diet!
A major advance in our understanding of the maintenance of life in subglacial environments was the recent discovery that H2 is produced by subglacial crushing. This is an important energy source for microbial food chains, because physical energy is transferred, via surface chemical (free-radical) energy, to biological activity and energy. Experiments show that around 10-20 nmol H2 is produced per gram of crushed rock after 120 hours (Telling et al., 2015). Even if they sound very small, these concentrations are significant since only sub-nanomolar concentrations of H2 are required to sustain microbial growth near 0°C (Hoehler, 2004). Hydrogen is utilized by many types of microbes, and is generated abiotically via the interaction of silica surface radicals with water.
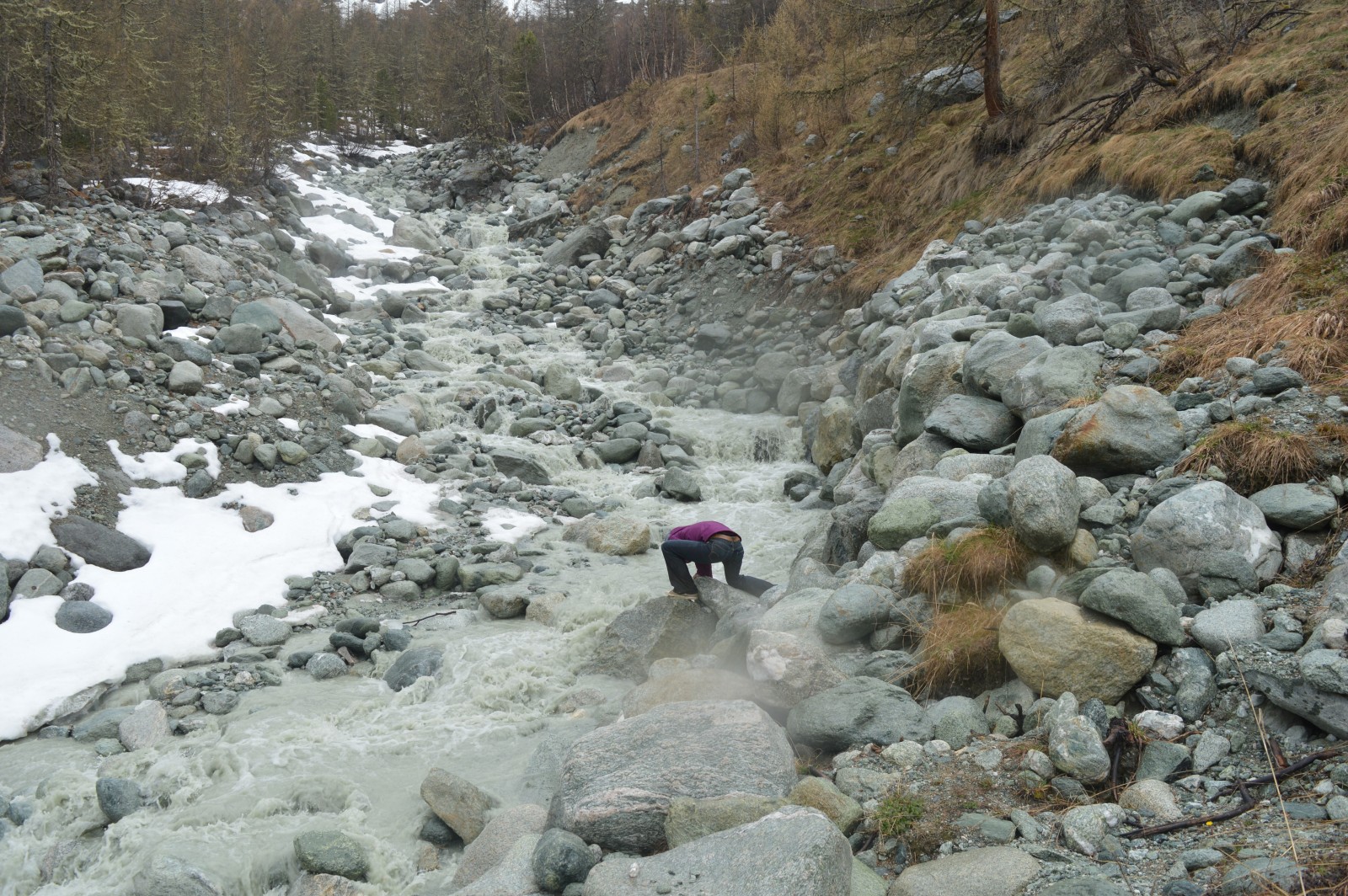
Next time you look at an otherwise dull grey meltwater stream draining a glacier, think of the crushing and the hydrogen that has been liberated by glacier erosion. The grey coloration arises from suspended sediment concentrations of about 1kg/m3 of meltwaters, and the sediment is typically silt- to clay-sized [Credit: Martyn Tranter].
Production of H2 supports the base of microbial food webs in fault and hydrothermal zones. Therefore, it is no stretch to suppose that this could be the case beneath glaciers. The H2 production rate from experimental rock crushing exceeds that required to support measured rates of methane production in the upper centimetre of South Western Greenland subglacial sediments. Additionally, rates of methane production in these subglacial sediments increased 10 times with the addition of excess H2 at 1°C (Stibal et al., 2012). A range of aerobic and anaerobic bacteria thought to be capable of oxidizing H2 as a source of energy have been found in subglacial sediments. Adding H2 to subglacial sediments from Robertson Glacier provided compelling evidence that the non-biological H2 produced during rock crushing could provide the sustain H2-oxidizing microbes (Telling et al., 2015).
Subglacial Lake Whillans is the first Antarctic subglacial lake to be sampled via a direct access hole. Water and sediment from the lake contain microbial life (Christner et al., 2013). Examination and experimentation on these unique samples is currently ongoing at the University of Bristol, where we hope to show that even already heavily weathered sediment produces hydrogen and supports microbial ecosystems when crushed and wetted. Subglacial microbes really do have a crush on glacier erosion, but don’t say it with chocolate or flowers, say it with hydrogen.
Edited by Joe Cook and Clara Burgard
Martyn Tranter is a polar biogeochemist, resident at the Bristol Glaciology Centre, University of Bristol, UK. Contact Email: m.tranter@bristol.ac.uk.