In a global context, New Zealand’s small mountain glaciers often get overlooked and yet they are a beautiful part of New Zealand’s landscape. They are the water towers for the South Island and an essential part of its tourism, thanks to a few undeniable heroes (Frans Josef and Fox Glaciers), but sadly, they may not be as prominent in the future. In this post we review the state of modern glaciation in New Zealand, explain why glacier-lake interactions are important and why we need to turn to the past for answers.
Glaciers in New Zealand are losing mass…
New Zealand is home to over 3000 glaciers. However, their mass is quickly declining. New Zealand has one of the oldest and most continuous records of annual ice volume, provided by the End of Summer Snowline Survey, and pioneered by the late Trevor Chinn. Now undertaken by NIWA, aerial surveys on 51 index glaciers have been carried out every year for more than 4 decades (records began in 1977). The surveys document the snowline on the glaciers at the end of every summer, which give us a timeline of glacier-climate interaction. Glacier snowlines, also known as Equilibrium Line Altitudes (ELAs), provide a direct measurement of a glaciers health. They record how much of the previous winter’s snow remains at the end of summer, contributing to long-term glacier ice accumulation. The higher the ELA, the less winter snow remaining, indicating the glacier has shrunk. If the ELA is lower, a larger amount of winter snow remains and the glacier has increased in size. An almost continuous trend from this 40 year archive reveals that glaciers in New Zealand are retreating rapidly – they are getting shorter and losing mass. The significant retreat of Southern Alps glaciers has accelerated over the last decade and recent studies have found that a third of total ice volume has been lost since records began.
…leading to the development of proglacial lakes
The formation of proglacial lakes in mountainous regions, specifically those in contact with the ice margin, is one of the consequences of glacier recession. As a glacier margin retreats, meltwater is impounded in the topographic low between the ice front and the abandoned moraine ridges. The increasing number and size of proglacial lakes is one of the most visually obvious effects of present deglaciation in New Zealand. Most of the existing ice-contact lakes in New Zealand formed when glaciers began to recede from large moraine ridges constructed during the Little Ice Age (LIA), about 150 years ago.
Proglacial lakes currently represent 38 % of the total number of all lakes in New Zealand, and over a third of the country’s perennial ice is contained within lake-calving glaciers. Back in the early 1970s, the Tasman Lake did not exist and the glacier terminated against its outwash sediments. Today, just 40 years later, it now terminates in a large proglacial lake that is more than 8 km long, and over 200 m deep (Figure 1). Since the lake formed, the Tasman Glacier has retreated at an average of 180 m per year and is now in a state of rapid recession.
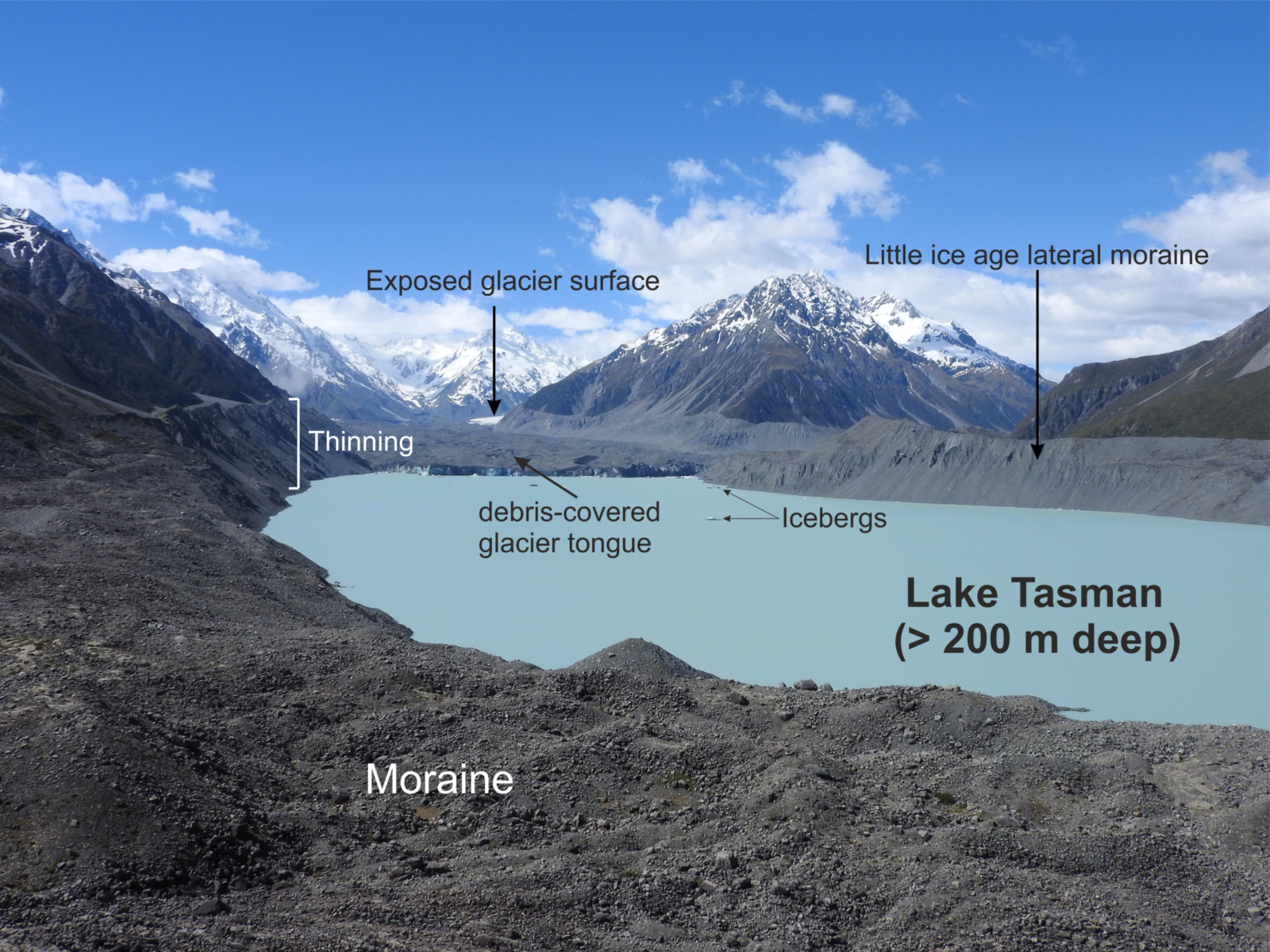
Figure 1. The Tasman Glacier and its associated proglacial lake. Don’t be fooled by how thin the glacier terminus looks from this perspective, the ice cliff is 50 m tall. The lower part of the glacier is heavily debris-covered but you can see the exposed glacier surface at the head of the valley [credit: Jenna L. Sutherland]
Lake formation is strongly linked to glacier dynamics (Figure 2). The depth of water at the ice margin determines:
- the distance underneath the glacier that water can travel
- the rate at which ice calves (or breaks off) from the terminus
Together, these factors increase the speed of ice flow and increase mass loss from the glacier system. The relatively warmer water of the lake compared to the glacier ice also causes thermally-induced melting. An ice-marginal lake can therefore cause glacier margins to fluctuate back and forth, which in turn can cause the speed of the glacier and its mass balance to become partially separated from the climatic signal.
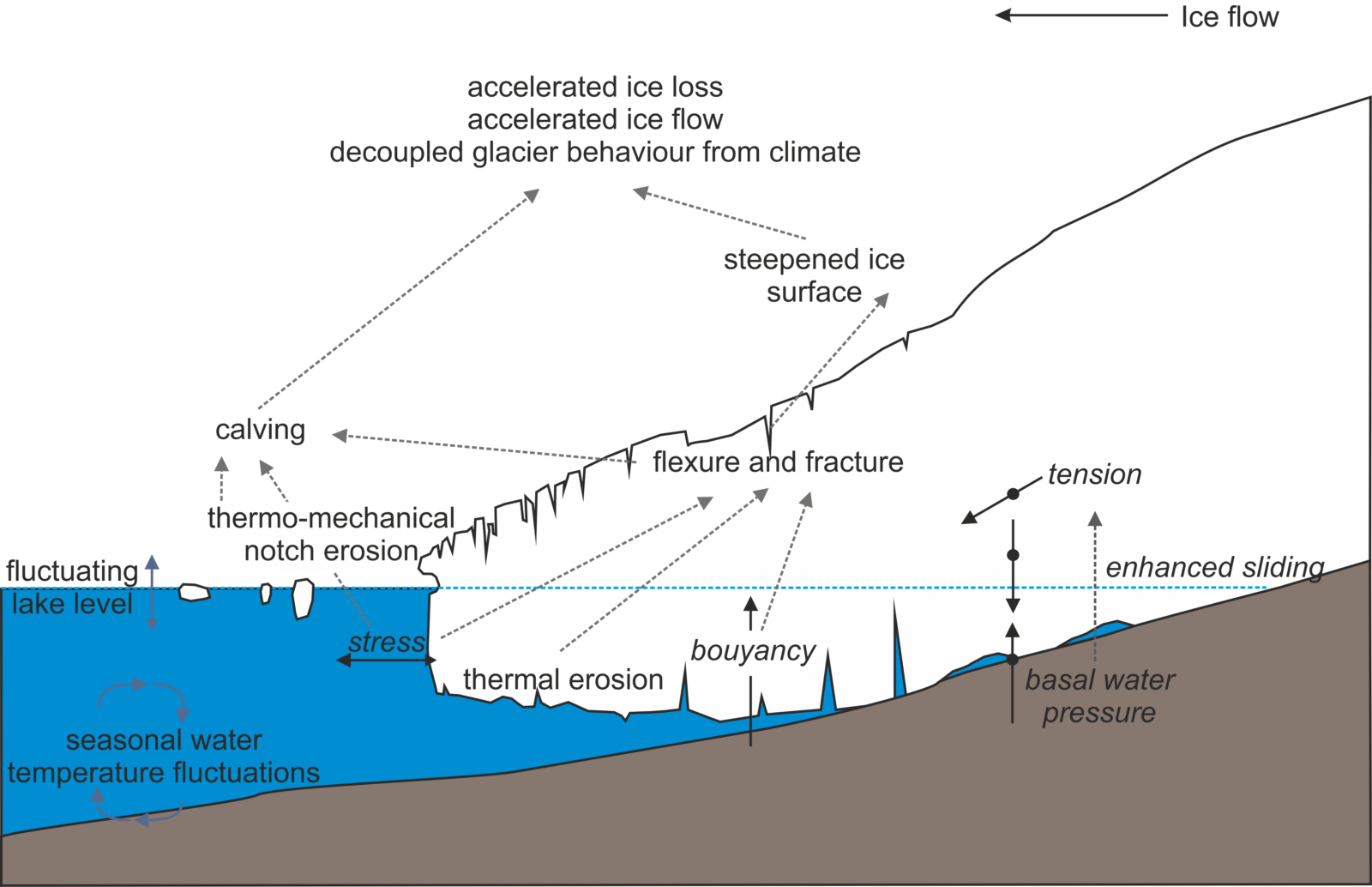
Figure 2. Interactions between the glacier and its proglacial lake. Forces acting upon the glacier are shown in italicised text and with black arrows. The processes between the lake and the glacier are in black text and grey dashed lines [Credit: Jonathan Carrivick, modified from Carrivick and Tweed, 2013]
New Zealand is witnessing unprecedented glacier recession together with lake expansion. It is easy to link one to the other, but the relationship between ice, the development of the lakes, and climate are much more complicated than this. The shift from a land-terminating to a lake-terminating glacier is a defining point in deglaciating environments. This transition represents a threshold and/or tipping point that is critical for the future evolution of the glacier and therefore crucial for us to understand. Could such glaciers become unstable and catastrophically collapse? Has this happened before? Where and what is this threshold? The current state of knowledge in New Zealand lies in monitoring temporal and spatial evolution of both glaciers and their proglacial lakes. Despite the remarkable record, the short duration of observations in New Zealand (just over 40 years) means that it is difficult to differentiate between natural cycles and occurrences, and dynamic behaviour that is beyond the norm. The answer to such questions lies in turning to the palaeo-record to find out how the Southern Alps ice field has behaved over the last few thousand years or more. It is vital to determine what thresholds control glacier-lake behaviour, and whether these have been crossed in the past. By gaining a deeper understanding of past processes, rates of change, thinning and retreat, as well as previous temperatures and environmental conditions, we will be better placed to understand how the Southern Alps could behave in the future.
Using the past to better understand the future….
The Quaternary record in New Zealand bears witness to the existence of proglacial lakes associated with retreat since the Last Glacial Maximum (LGM; the period between 21,000 and 18,000 years ago). During the LGM, the ice extent and volume was much larger; outlet glaciers advanced beyond the present coastline along the west coast of the South Island (Figure 3). Just like we see in the modern day, the glaciers receded when temperatures began to warm and meltwater ponded, forming large and deep proglacial lakes in contact with the ice margin. Past glacier behaviour in New Zealand has been derived from mapping and dating former ice extents. The maximum extent of ice during previous glaciations is now well constrained, allowing us to determine the speed of glacier recession and thinning. A study has shown that rapid ice recession in the first few millennia saw glacier trunks thin by at least several hundred metres, with implied terminal recession by as much of 40% of the overall glacier length.
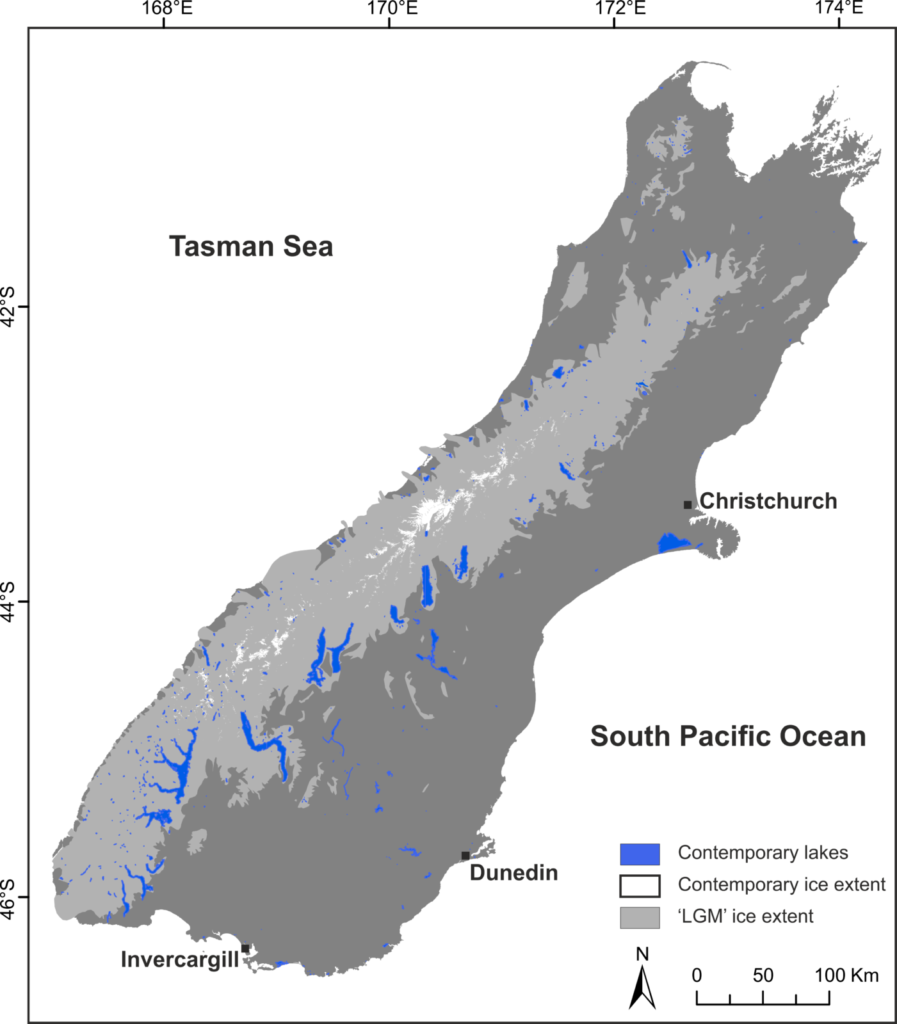
Figure 3. The South Island of New Zealand with modern-day glaciers mapped in white and LGM ice extent in light grey. Present day lakes (blue) would have once been in contact with retreating ice margins [Credit: Sutherland et al., 2019]
It has been suggested that the retreat of glaciers in the Southern Alps, immediately after the LGM, was relatively rapid not only because of a warming climate, but also because of the widespread formation of large proglacial lakes accelerating recession. However, despite knowing that large proglacial lakes existed, we have no understanding as to what effect they had on glacier retreat and how much influence these proglacial lakes had on glacier dynamics in combination with climatic warming. The interactions between proglacial lakes and glacier dynamics have not yet been quantified. This is largely a consequence of poor accessibility to modern glacier-lake margins but also because proglacial lakes are currently ignored in ice sheet models (Figure 4). They are treated as an entirely separate component, or, as is more often the case, not at all.
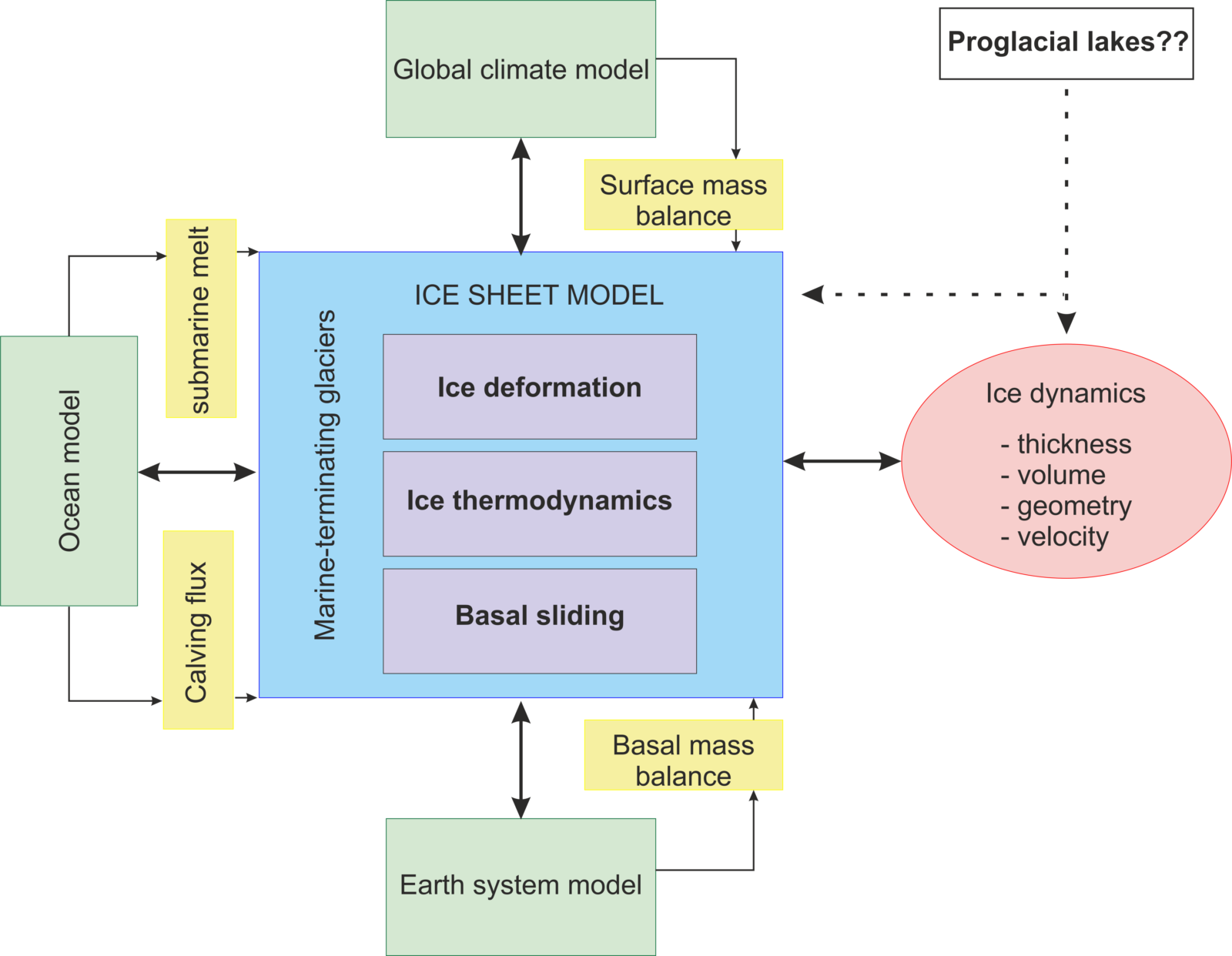
Figure 4. Main components of most ice sheet models (blue), often coupled to other models (green), e.g. ocean and atmosphere models. Note the absence of proglacial lakes as a component of the ice sheet model despite their importance in influencing glacier dynamics [Credit: Jenna. L Sutherland]
…But not without some computer modelling too!
Owing to numerous studies that have dated the recession of ice since 18,000 yrs ago we have a pretty good idea that glacier recession was fast. However, as a concern for the future, what we are more interested in is constraining previous ice volumes and, more specifically, the mechanisms of loss. As well as rates of change, we also need to understand processes of change and how the ice evolved through time. In order to examine and better understand the external (or internal, as the case may be) forcing that drove this rapid recession, we need to resort to relating glacier and climatic changes in numerical ice sheet or glacier simulations.
The physics that govern a glaciers mass balance (the difference between snow gain and snow melt) as well as ice flow are complex. The equations depend on ice thickness, speed, ice and air temperature, elevation, as well as many other factors. Fortunately, these relationships are reasonably well understood and provide the basis of many numerical ice sheet models. We then feed the model with input data, such as topography and past climate, to drive a numerical ice sheet simulation. This enables us to investigate detailed mechanisms driving ice sheet change, such as those between a glacier and its lake and we can become confident in such models when they provide a good fit to the geological record (what we see on the earth’s surface).
The future
Understanding the formation and evolution of proglacial lakes and their outlet glaciers through time can provide insights into the behaviour of glaciers and ice sheets to help us anticipate some of the impacts of present and future deglaciation. Although my research is concerned with just one small valley glacier in a specific region, it is a small step towards a wider understanding of the glacier-lake interaction phenomenon. Of course, every proglacial lake is different, just as every mountain glacier is, but if we can get a handle on what effects a lake had on its glacier in the past, the importance of proglacial lakes might be realised for other regions and more seriously considered when it comes to interpreting glacier response to climatic change.
Further Reading
- Tweed, F. S., & Carrivick, J. L. 2015. Geology Today. Deglaciation and proglacial lakes
- Image of the week – Looking to the past for answers
- AntarcticGlaciers.org – Glacial Lakes
- AntarcticGlaciers.org – Numerical ice sheet models
Edited by Andy Emery
Jenna Sutherland is a final year PhD student in the Department of Geography at the University of Leeds, UK. Her research is focused on the interaction of proglacial lakes and their outlet glaciers during the Last Glacial Maximum in New Zealand, specifically by simulating the presence of proglacial lakes in a numerical ice sheet model and relating these experiments to the sediment-landform record. Her broader interests lie in palaeo-glacial environments. She tweets from @Jennalo13