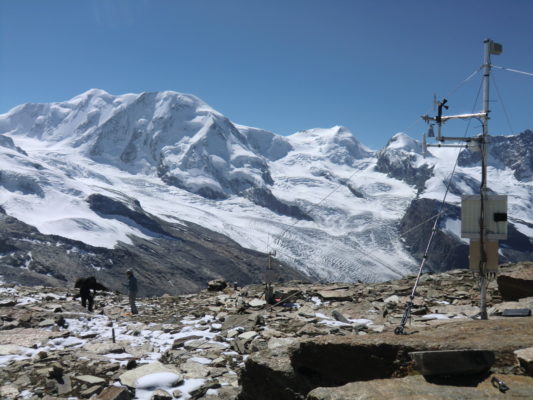
Through the eyes of a researcher studying mountain processes in the European Alps in the context of a global warming climate, let’s discover how geophysical methods help to better understand frozen ground!
Permafrost defines a thermal state, i.e. permafrost is soil, sediment, or rock that remains at or below 0°C during at least two consecutive years. As permafrost is only defined by its temperature, it may contain ice as a mixture of liquid water and ice, or be dry (without any water molecules, i.e. no ice and no liquid water). Compared to glaciers or snow, the presence of permafrost is much more difficult to assess, as it is underground, and so by definition invisible (as you can see, or not, in Photo 1). Research on permafrost very often implies measuring and monitoring ground temperatures. But our knowledge of the ice content of the ground (also called ground ice) usually remains poor with solely temperature information.
Why is monitoring ground temperatures not enough?
Regularly measuring ground temperatures from boreholes is a standard practice to monitor the evolution of permafrost, including its degradation. The onset of freezing can be determined precisely through temperature monitoring. However, when permafrost starts to thaw (i.e. melting the ice), it starts with a phase change from the ice phase to the water phase. And during that phase change, the temperature remains constant at about 0°C. So the temperature offers no information about the amount of ice or the amount of liquid water (i.e. ice-to-water ratio) during the phase change. As a consequence, freezing and thawing processes cannot be analysed through temperature measurements alone.
Why do we care about ground ice content?
The amount and distribution of ground ice both influence the stability of a terrain through controlling the mechanical properties of the terrain. Thawing permafrost (i.e. melting the ice) affects mountain slope stability and can trigger rock falls. With global warming, increasing temperatures and associated thawing processes, can threaten the stability of cliffs, when the fractures within them are sealed with ice (cf. this Cryoblog post).
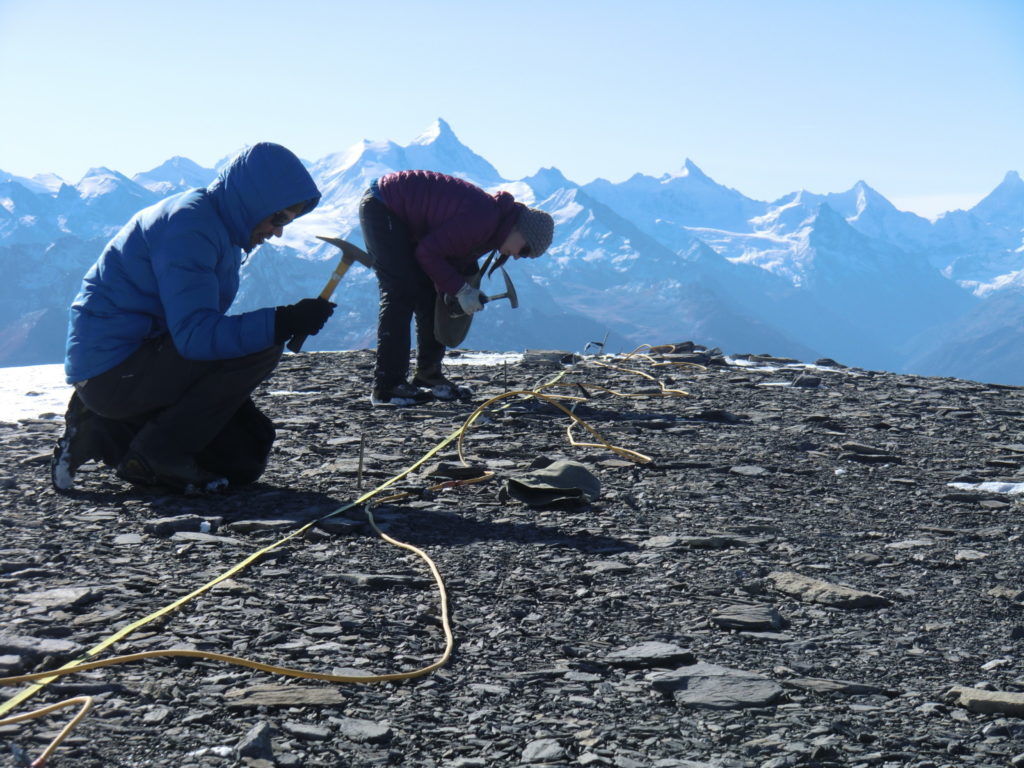
Photo 2: Installation of a geophysical profile (Electrical Resistivity Tomography) in the Swiss Alps to image the subsurface and find out where ground ice is present. To know more about this technique from a poetry point of view, see this Cryoblog post. Photo credit: Coline Mollaret
How do researchers study permafrost then?
Drilling into permafrost and recovering core samples allow a detailed analysis of the ice content. Indeed, cores provide high resolution data, but low spatial coverage (you only get the-one-core, as drilling is expensive). In addition to measuring the ice content directly, several geophysical methods can be used to estimate qualitatively or quantitatively the amount of ground ice. These geophysical methods have a wider spatial coverage, lower cost, but also a lower resolution due to the technique (e.g. electrical methods, seismic methods, ground penetrating radar, and gravimetry). These geophysical methods allow to image the ground’s geophysical properties (Photo 2). The ground’s geophysical properties are related to its composition such as its water content and ice content. For instance, electrical resistivity measurements (how much the ground lets electricity through) are very sensitive to the water-ice phase change (as ice is an electrical insulator in contrast to liquid water, which is an electrical conductor).
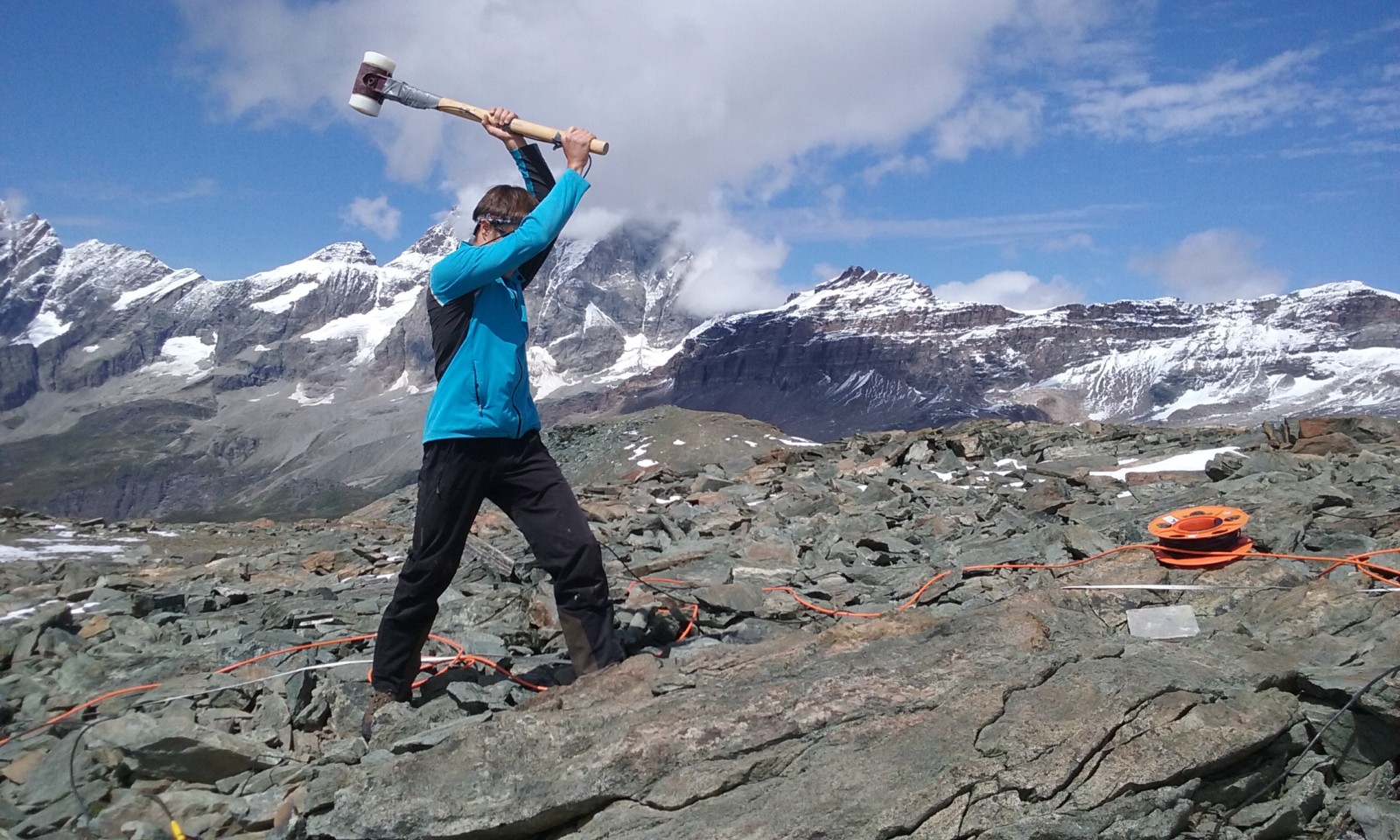
Photo 3: Hammering the ground to produce seismic waves (similarly to small earthquakes) in order to image the seismic properties of the ground. Photo credit: Coline Mollaret
Which new method did we develop to estimate ground ice?
The new technique, inspired by the four-phase model by Hauck et al. (2011), has recently been developed by Wagner et al. (2019), quantifies the composition of the ground from several geophysical data sets. It uses both P-wave velocity measurements illustrated in Photo 3 (P-waves are a type of seismic waves that travel faster than all the other seismic waves) and electrical resistivity (cf. this Cryoblog post) to quantify the phases of water, ice, air, and rock (called hereafter the four phases).
This new technique is called a “petrophysical joint inversion”. Let’s decompose this into its components. “Petrophysical” because it is based on a petrophysical model (relating the four phases to geophysical properties, such as the electrical resistivity and the P-wave velocity). “Inversion” implies that the technique starts from the effects observed (measurements at the ground surface, cf. Photo 2) to derive the causes (in the underground, e.g. at 0-20 m depth). And “joint” because the inversion scheme uses the specific sensitivities of two geophysical data sets (electrical resistivity and seismic velocity) at the same time, which reduces the uncertainties of the results.
This new petrophysical joint inversion is able to resolve ice-poor to ice-rich conditions (which is a significant improvement!) and leads to ground ice estimations in good agreement with available observations (Mollaret et al., 2020). The ground ice content was examined in different alpine sites and found to be 5 to 15% in bedrock, 20 to 40% at a talus slope (pile of rocks that accumulates at the base of a slope) and up to 95% at rock glaciers. Another significant advantage of this joint inversion technique is that it can be applied on all existing geophysical data sets and be repeated over time to quantify the ground ice loss and its variability over time and space in the context of global warming.
Permafrost and especially the ground ice content are key “thermometers” of climate change and can thus allow us to understand changes occurring in mountainous regions.
Further reading
- Mollaret C., Wagner F.M., Hilbich C., Scapozza C. and Hauck C. (2020) Petrophysical Joint Inversion Applied to Alpine Permafrost Field Sites to Image Subsurface Ice, Water, Air, and Rock Contents. Frontiers in Earth Science. 8:85. https://doi.org/10.3389/feart.2020.00085
- Wagner F.M., Mollaret C., Günther T., Kemna A. and Hauck C. (2019) Quantitative imaging of water, ice and air in permafrost systems through petrophysical joint inversion of seismic refraction and electrical resistivity data, Geophysical Journal International, Volume 219, Issue 3, Pages 1866–1875. https://doi.org/10.1093/gji/ggz402
- Hauck, C., Böttcher, M., and Maurer, H. (2011) A new model for estimating subsurface ice content based on combined electrical and seismic data sets, The Cryosphere, 5, 453–468, https://doi.org/10.5194/tc-5-453-2011
- Cryoblog post on cliff stability
- Cryoblog post on Electrical Resistivity Tomography
- Cryoblog post on electrical resistivity
Edited by David Docquier and Marie Cavitte
Coline Mollaret is a geophysicist researcher who just finished her PhD at the University of Fribourg, Switzerland. She investigates mountain permafrost through geophysical methods in relation to the climate change. She is particularly interested in geophysical monitoring and processing methods such as joint inversions to quantify the composition of alpine ground. Contact email: coline.mollaret@unifr.ch