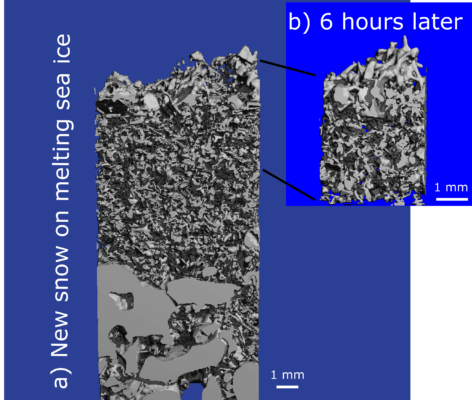
When I was a student, Martin told me matter-of-factly that snow is hot. I probably looked as baffled as I felt (and you are probably doing the same). Were we talking about the same thing? Continue reading to discover why snow is hot!
Discovering that snow is hot
So why is snow hot? Most snow under Earth’s environmental conditions has a homologous temperature Th close to 1. The homologous temperature is expressed as the ratio of the temperature of a material (in K) and that material’s melting temperature. The term is used to determine the steady-state creep rate in crystalline materials. Steady-state creep is a material’s ability to move slowly under persistent mechanical stress and is a function of the material’s homologous temperature, amongst other factors. This is relevant for many applications in material science and natural processes, such as lava flows and, more importantly for cryosphere scientists, for glacier flow and snow deformation.
So back to snow and ice: at -10°C, snow’s Th is 0.96; for snow at -30°C, Th is 0.89. At -50°C, which is quite cold for Earth, Th is still 0.82, so ice crystals will happily deform without much difficulty. For granite to behave similarly to snow and ice at -10°C, it would need to be at ~1200°C, so essentially hot lava. Because of snow and ice’s high Th, geologists use ice as an analog for rock-forming minerals (Wolson et al. 2014, Fang et al. 2022) because it deforms fast and deforms at temperatures and pressures that are attainable without heating your lab to 1200°C. Would you ever have guessed that frozen water helps study the behaviour of rocks?
Hot snow means fast sintering
Because of the high Th, snow (and ice) sintering has the fastest rate of any other earth material at similar pressures and temperatures (Szabo and Schneebeli, 2007). Sintering is the process of “gluing” particles together by heat and pressure without melting. Figure 1 shows an example of fresh snow sintering during the MOSAiC expedition, as seen by microCT (micro-computed tomography). The left image shows new snow, and the right image shows the snow at the same location ~six hours later, where significant sintering occurred despite negative air and snow temperatures. In addition, snow also undergoes rapid microstructural changes caused by the exchange of water vapor within the snowpack, which we call snow metamorphism. The consequence of these fast-paced changes is that as soon as a snowflake hits the ground, it can barely be recognized as a snowflake.
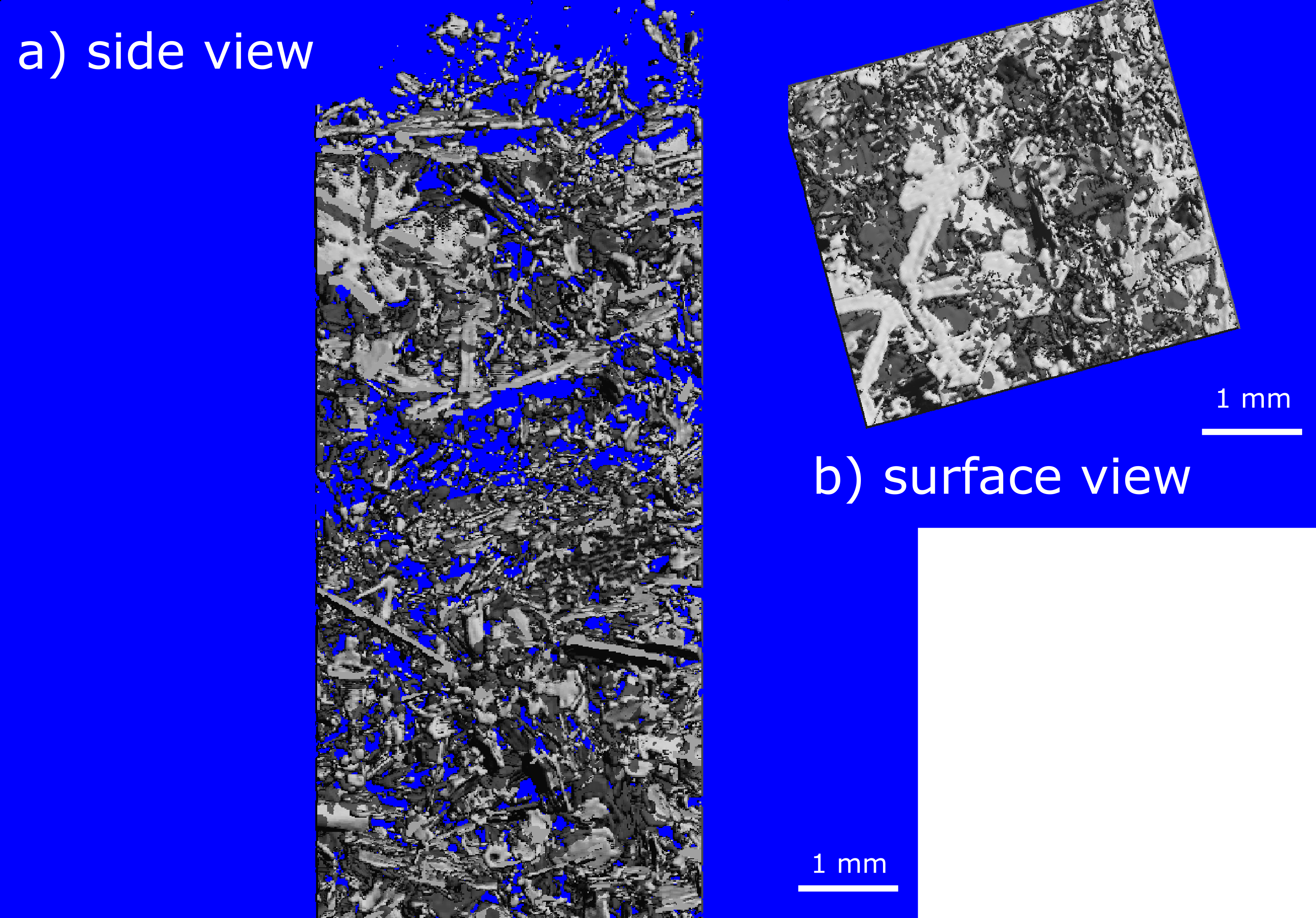
Figure 2: MicroCT images of fresh snow on the ground in side view (a) and surface view (b) during the late summer phase of the MOSAiC expedition. A few snow crystals can still be recognized, but they are rare.[Credits: Ruzica Dadic]
So, now you know that snow is super hot, and when you see a lovely perfect snow crystal, it really is ephemeral.
But why do we care? Well, snow cover evolution is the single largest annual change observed on Earth’s surface. And snow is one of the most insulating materials on Earth and acts as a barrier preventing heat exchange between the surface, the atmosphere (and in the case of sea ice, the ocean). Snow is also one of the most effective natural reflectors on the planet and thus influences how much energy is absorbed on the Earth’s surface and how much is reflected into space. And last but not least, because snow is an extremely effective seasonal water reservoir, it affects freshwater availability and quality of life in large areas of our planet (e.g. Simpkins 2018).
Further reading:
- Wilson et al. (2014). Microstructure and fabric development in ice: Lessons learned from in situ experiments and implications for understanding rock evolution. Journal of Structural Geology 61:50– 77.
- Fan et al. (2022). Using misorientation and weighted Burgers vector statistics to understand intragranular boundary development and grain boundary formation at high temperatures. Journal of Geophysical Research: Solid Earth 127:e2022JB024497.
- Szabo & Schneebeli (2007). Subsecond sintering of ice. Applied Physics Letters 90:151916.
- Simpkins. Snow-related water woes. Nature Clim Change 8:945.
Edited by Loeka Jongejans and Giovanni Baccolo
Ruzica Dadic is a senior research fellow at the Antarctic Research Centre, Victoria University of Wellington, New Zealand. She is currently a visiting fellow at the WSL Institute for Snow and Avalanche Research SLF in Davos, Switzerland. Ruzica works toward understanding the role of the physical properties of snow and ice on global climate. Currently, she works on snow processes in Arctic and Antarctic sea ice. (Photo credit: Mario Hoppmann)
Martin Schneebeli is a senior researcher at the WSL Institute for Snow and Avalanche Research SLF in Davos, Switzerland. Martin has pioneered the experimental investigation of snow’s physical properties for 30 years, and he is interested in all physical and geological processes occurring in the snowpack, and in different environments. He works toward applying quantitative stratigraphy to larger-scale processes, such as remote sensing, avalanche formation, and climate research. (Photo credit: Luzia Schär)