As scientists, we often encounter errors in our own work and in the work of others. As modelers, we call these errors “bugs”. Of course, they are not actual insects, but they definitely keep us awake from time to time. Even though everyone is aware of their existence, we rarely discuss them in a scientific context. In today’s post, I bring to you the work and journey of a snow scientist, Dr. Cécil ...[Read More]
Did you know that snow is hot?
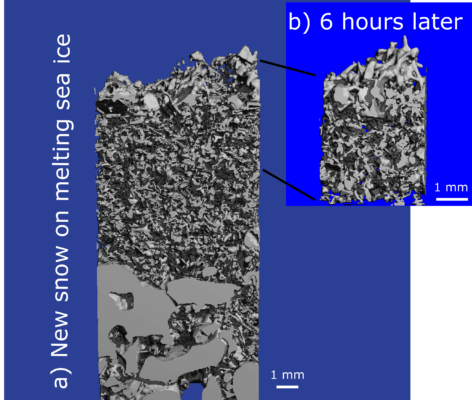
When I was a student, Martin told me matter-of-factly that snow is hot. I probably looked as baffled as I felt (and you are probably doing the same). Were we talking about the same thing? Continue reading to discover why snow is hot! Discovering that snow is hot So why is snow hot? Most snow under Earth’s environmental conditions has a homologous temperature Th close to 1. The homologous temperatu ...[Read More]
Image of the Week – 5th Snow Science Winter School
From February 17th to 23rd, 21 graduate students and postdoctorate researchers from around the world made their way to Hailuoto, a small island on the coast of Finland, to spend a week learning about snow on sea-ice for the 5th Snow Science Winter School. The course, jointly organized by the Finnish Meteorological Institute and the Swiss Federal Institute for Forest, Snow and Landsca ...[Read More]
Image of the Week — Orange is the new white
On 22 March 2018, large amounts of Saharan dust were blown off the Libyan coast to be further deposited in the Mediterranean, turning the usually white snow-capped Mountains of Turkey, Romania and even Caucasus into Martian landscapes. As many people were struck by this peculiar color of the snow, they started documenting this event on social media using the “#orangesnow hashtag”. Instagram and t ...[Read More]
Image of the Week — Listening to the Snow
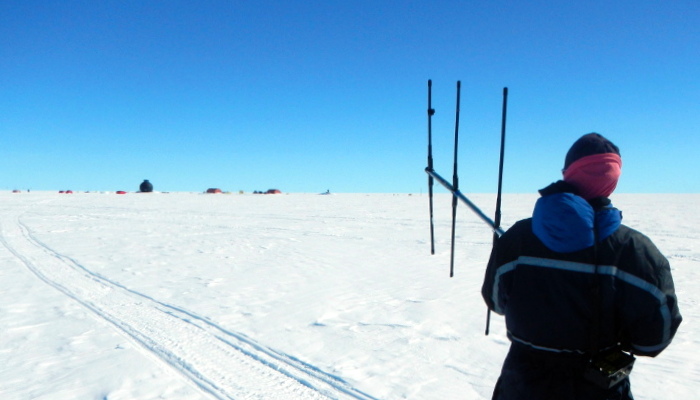
When working in the middle of an ice sheet, you rarely get to experience the amazing wildlife of the polar regions. So what are we doing hundreds of kilometres from the coast with an animal tracker device? We are listening to the snow of course! It is not crazy; It is what Image of the Week today is all about! Going Wireless In June 2016, Liz Bagshaw and I travelled to the EGRIP (East Greenland Ic ...[Read More]
Image of the Week – The Journey of a Snowflake
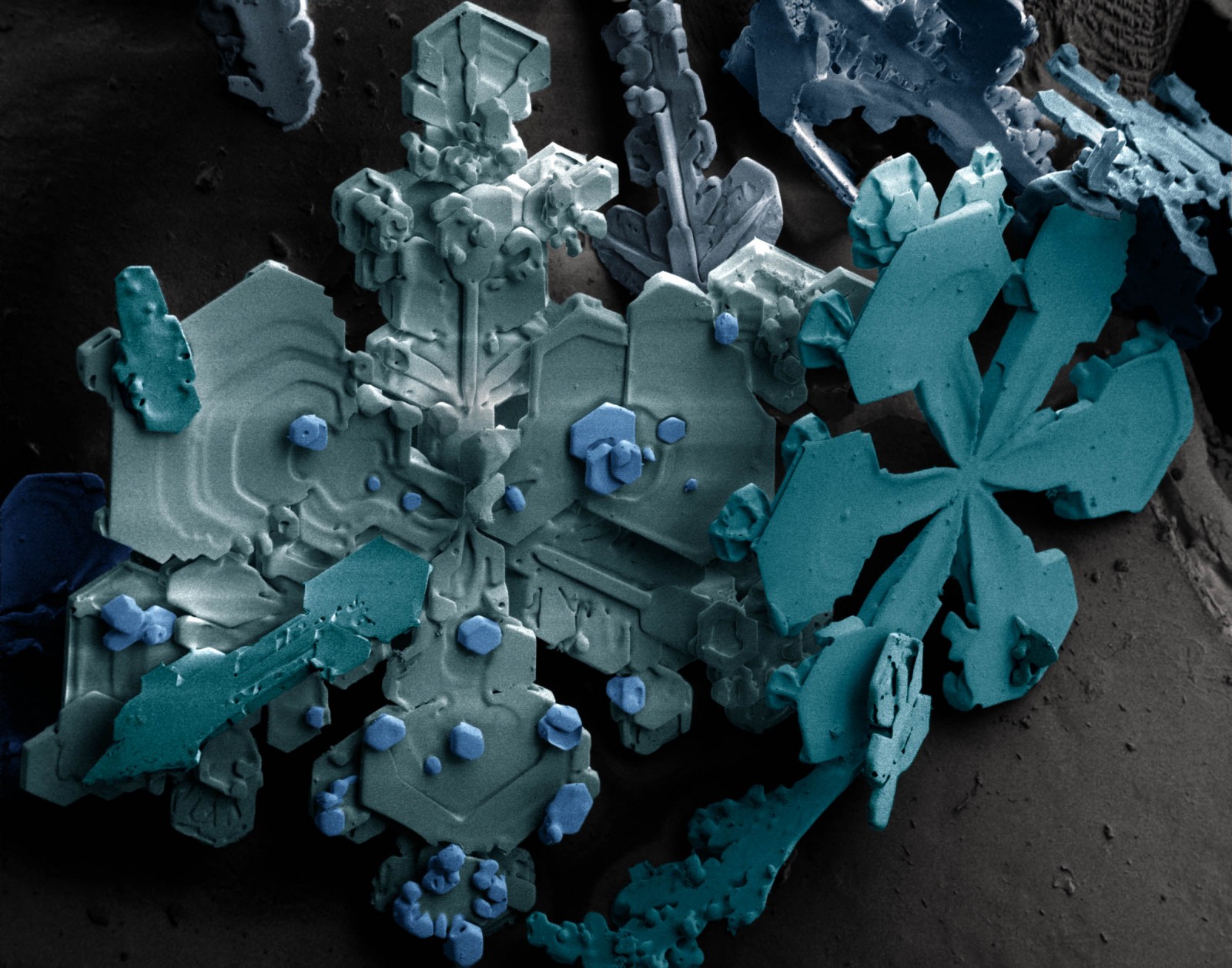
You remember last winter, when everything was white and you had so much fun building a snowman with your friends? What you see on this image above, is what you would see, if you took a tiny tiny piece of your snowman and put it under a low-temperature scanning electron microscope (SEM). The colours are called “pseudo colours”, they are computer generated based on the number of electron ...[Read More]
Image of The Week – A Game of Drones (Part 1: A Debris-Covered Glacier)
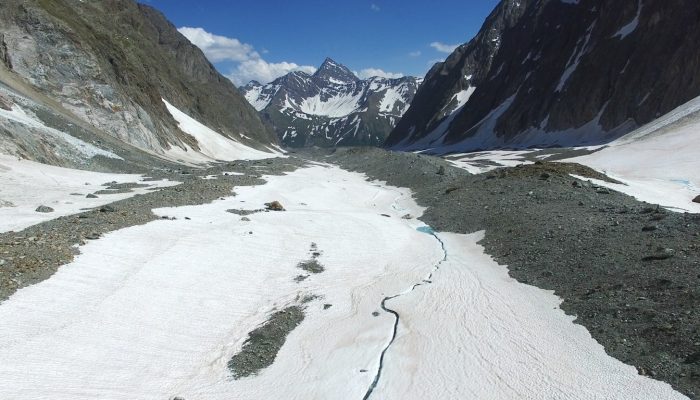
What are debris-covered glaciers? Many alpine glaciers are covered with a layer of surface debris (rock and sediment), which is sourced primarily from glacier headwalls and valley flanks. So-called ‘debris-covered glaciers’ are found in most glacierized regions, with concentrations in the European Alps, the Caucasus, Hindu-Kush-Himalaya, Karakoram and Tien Shan, the Andes, and Alaska and the weste ...[Read More]
Image of the Week: Changes in Snow Cover
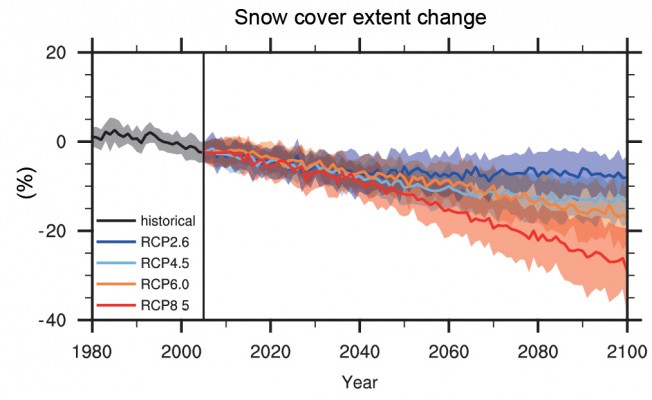
Who is dreaming of a white spring? In daily life we might be more interested in the chances of a white Christmas, but the amount of snow-covered ground in the spring is a very good indicator of climate change. The figure above shows the projected change in snow cover extent in the Northern hemisphere in March-April according to different future scenarios (i.e. Representative Concentration Pathways ...[Read More]