Climate models help us understand processes occurring in and between atmosphere, oceans, land and ice-covered regions of our planet. One important process impacting all of us is sea level rise, and the ice sheets of Antarctica and Greenland (currently losing more and more ice) play a crucial role in future sea level rise projections. Even though climate models can be very complex and include many components, ice sheet models are just slowly starting to be directly integrated into climate models. This article explains why that’s the case and why coupling ice sheet models to climate models can be tricky.
What is a climate model?
All climate models are based on sets of equations to capture processes in the Earth system (Fig. 1) and come with various complexities. Their coverage can vary between distinct regions of the world (like the Mediterranean Sea) to the entire globe.
Climate models consist of different components (like atmosphere, ocean, land and cryosphere), which are developed separately but are programmed to talk to each other and exchange needed data. The communication and variable exchange between different components of a climate model is called coupling. Coupling can be done either as one-way (e.g. the ice sheet model receives the amount of snowfall calculated by the atmospheric model, but the atmospheric model does not receive any updated variables from the ice sheet model) or two-way (the atmospheric model, in addition, receives information about the new ice surface elevation from the ice sheet model. It can thus calculate again the amount of snow that will fall onto the ice sheet and then send back the new amount of snowfall to the ice sheet model, and so on).
How it all started
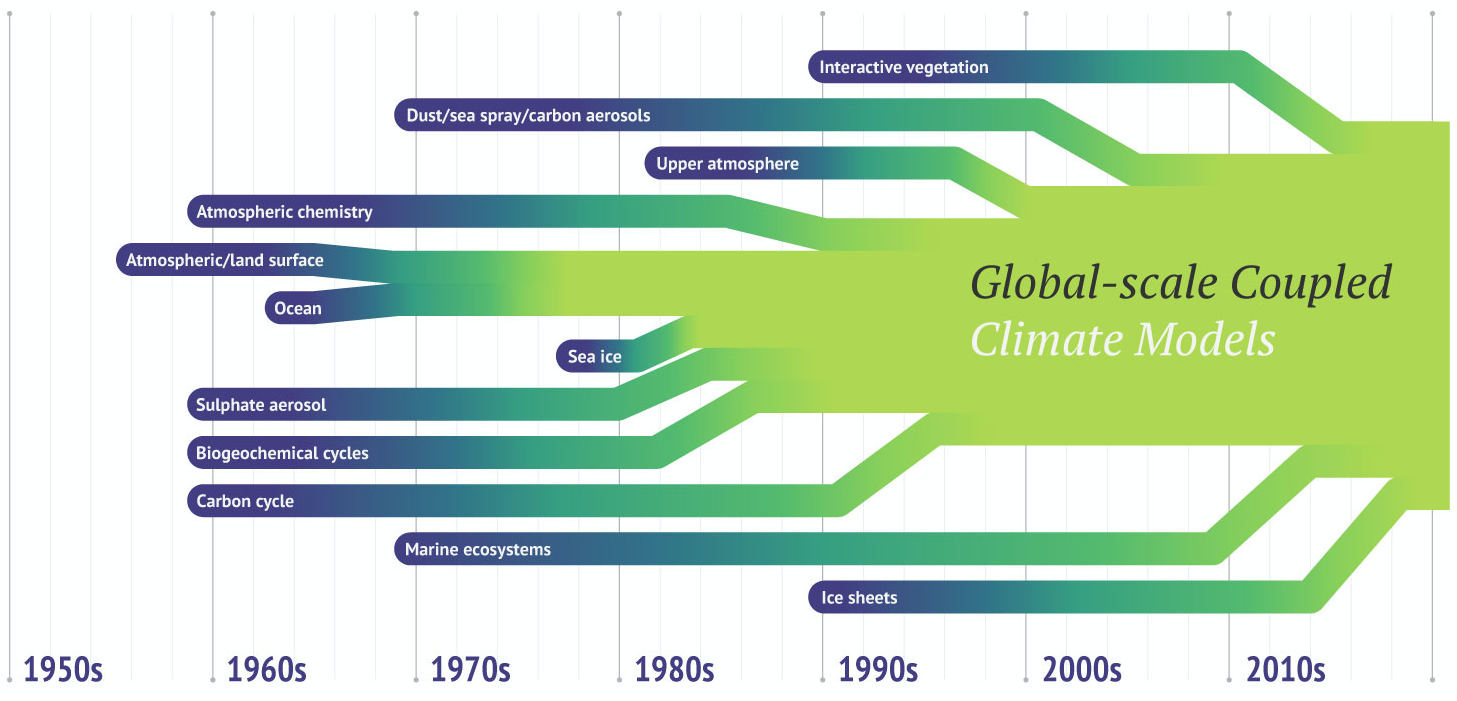
Figure 2: Historical evolution of the components of climate models [Credit: CarbonBrief: Q&A How do climate models work?]
Ice sheet models are relatively young compared to climate models. Already in the 1950s and 1960s started the development of atmospheric and ocean models (see Fig. 2). Those models started simple, but with the increasing amount of data/measurements and computers slowly improving, the first coupling of atmospheric and ocean models could already be done in 1975. From there, climate models became better and more complex. At that time, scientists studied many aspects of the climate system, but ice sheets were thought to be stable and only to change on time scales of thousand years, not having a big impact on the climate. (Which was true at that point in time: Greenland’s and Antarctica’s mass balance was quite stable in the 1970s and 1980s.)
Furthermore, there were only very few science expeditions to the Arctic and Antarctica to collect data and satellites were only slowly starting to deliver sparse, low resolution pictures making it hard to study those remote, icy places (see this post and this post). While sea ice was early on included as an important boundary layer between atmosphere and ocean, the rest of the cryosphere (mountain glaciers, Greenland, Antarctica), was (and most of the time still is) assumed as a consistent ice mass not changing in size. Hence, a usual procedure is that ice sheet models use climate model data (like the amount of snowfall) and simulate the contribution of ice melting to sea level change, but do not give feedback directly to climate models. Even though those changes have an impact on the climate (see this post).
Ice sheet models started to pick up in the 1990s when the atmospheric and ocean models were already well established. Like atmospheric and ocean models, ice sheet models first had to evolve and improve, including more and more local processes and investigating if and on what time scales those processes would be important. Ice sheet model intercomparison projects (like SeaRISE, ice2sea, ISMIP) helped to identify important ice sheet processes and differences between models to reduce errors in projections of sea level change. Only recently, ice sheet models got to a point where results are much better.
So, what’s the problem now?
Ice sheet models need climate data to capture the past and project the future evolution of ice sheets and glaciers. Therefore, one-way coupling started early on, especially on paleo time scales, where ice sheet models could change for example the amount of snow fall feeding the ice sheet through time as a number directly calculated by climate models.
However, climate models in the 1990s could easily have a resolution (size of the grid cells in the model) of ~200-500 km, while ice sheet models have a resolution between few hundred meters to ~20 km, and this difference in resolution had to be overcome. Over time, climate models have increased their resolution (so smaller distance between grid points of the model) and techniques have been developed to simplify the data transfer between ice sheet and climate models.
Similarly, there are differences in the temporal resolution between ice sheet and climate models, meaning how often the model calculates its processes during the same time. A climate model is simulating changes about every 30 minutes – or even more often. An ice sheet model usually would have time steps between days to several months. This difference can be explained by the type of processes each model tries to simulate: fast, short-term changes in atmosphere or ocean, or slow, but persistent processes on ice. Just imagine how you can be surprised by suddenly approaching rain cloud while you are hiking on a nice sunny day. In contrast, you can return every hour to the same glacier (maybe during your vacation in the Alps) and you won’t observe any changes on the ice. You might first see changes on the glacier when you come back after some days, weeks or months.
Due to these offsets in spatial and temporal resolutions, coupling can become a quite complex task, especially concerning the exchange of information (or variables) between the models. We won’t go further into detail here not to become too technical.
Next steps
There are already many one-way coupled studies, where only the ice sheet model receives data from the climate model during the simulation. Currently, some individual studies push two-way coupling approaches (both models exchange data) by using climate models together with ice sheet models (for example Ziemen et al., 2019, Seroussi et al., 2017, Goelzer et al., 2016, Calov et al., 2002).
An example for an ongoing coupling project is PARAMOUR, which investigates the feedbacks between atmosphere, land ice, sea ice and ocean around Greenland and Antarctica on decadal time scales. One of its research questions is whether the climate system will evolve differently (showing different feedbacks) if atmospheric, ocean and ice sheet models are coupled on relatively small regions, such as Totten Glacier (a glacier in Eastern Antarctica), or on the entire Antarctic Ice Sheet and Southern Ocean.
Another approach to improve coupling is to adapt climate models, for example by including the areas that are important for ice sheets (like the ocean beneath the floating ice shelves) into climate models.
Even further steps are taken by the Ice Sheet Model Intercomparison Project (ISMIP6, see blog), where selected climate scenarios (from CMIP6) are simulated over Greenland with a fully coupled atmospheric-ocean-ice model. Currently, the two climate models CESM2 and UKESM have those simulations running.
While coupling between ice sheet and climate models is still quite challenging, the science community is making progress on that topic with more and more projects helping to improve it. In the end, these efforts will allow to better understand the climate system and reduce the uncertainty in the projections of sea level rise.
Further reading
- Gettelman A., Rood R.B. (2016) Demystifying Climate Models. Earth Systems Data and Models, vol 2., Springer, Berlin, Heidelberg, doi:10.1007/978-3-662-48959-8_4
- CarbonBrief: How do climate models work?
- Goelzer, H., Robinson, A., Seroussi, H., van de Wal, R. (2017) Recent Progress in Greenland Ice Sheet Modelling. Curr Clim Change Rep 3, 291–302. doi:10.1007/s40641-017-0073-y
- Nowicki, S. M. J., Payne, A., Larour, E., Seroussi, H., Goelzer, H., Lipscomb, W., Gregory, J., Abe-Ouchi, A., and Shepherd, A. (2016) Ice Sheet Model Intercomparison Project (ISMIP6) contribution to CMIP6, Geosci. Model Dev., 9, 4521–4545, doi:10.5194/gmd-9-4521-2016
Edited by David Docquier and Vio Coulon
Konstanze Haubner is a post-doctoral researcher at the Université libre de Bruxelles (ULB) in Brussels, Belgium. She works on coupling ice sheet simulations with atmospheric and ocean models on Totten glacier (Aurora Basin, Antarctica) within the Belgian project PARAMOUR.