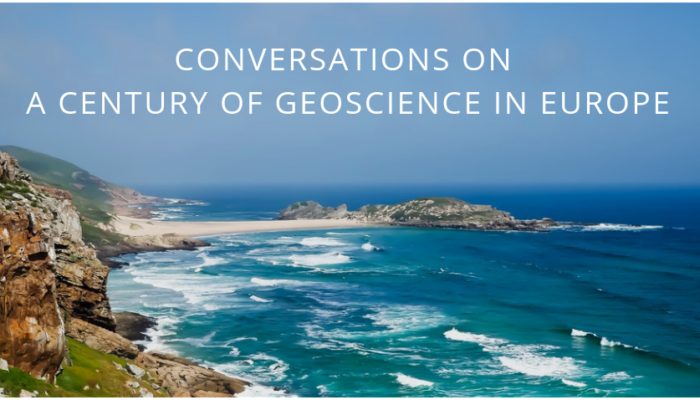
Over the last century, geoscientists have made incredible contributions to our understanding of the Earth, the solar system, and beyond. Inspired by the American Geophysical Union (AGU) and the International Union of Geodesy and Geophysics (IUGG) centennials, which are celebrated in 2019, we would like to highlight Europe’s role in shaping the geosciences and the great achievements of European geoscientists within the last century.
In this series of interviews, scientists reflect on the last 100 years of Earth, space and planetary sciences in Europe and share their perspectives on the future.
John Burrows: Professor of the Physics of the Ocean and Atmosphere and a Director of the Institutes of Environmental Physics and Remote Sensing at the University of Bremen
In your opinion, what are some of the biggest ways Europe and European scientists have shaped the geosciences within the last century?
The geosciences have evolved dramatically since the beginning of the 20th century, by building on the advances of our knowledge of physical chemistry, atomic and molecular physics, and biogeochemistry, coupled with improvements in how we measure physical parameters and chemical constituents (compounds and particles) in the Earth system. The invention of modern computers, pioneered initially by Allan Touring in the UK, coupled with advances in numerical mathematics, is another reason why the geosciences have blossomed. The accuracy of mathematical models, used to simulate and predict weather, the chemical composition of the atmosphere and climate, has continually improved in the past century.
European scientists played key roles in furthering our understanding of the Earth’s atmosphere and how it is influenced by natural phenomena and human activity. I begin with highlighting Swedish scientist Svante Arrhenius, who not only revolutionised chemistry by proposing the Arrhenius formula for the temperature dependence of reaction rate coefficients, but also made the first estimates, which showed that increasing atmospheric carbon dioxide increased atmospheric temperatures. Similarly, breakthroughs in atomic and molecular spectroscopy by a number of European and Europe-based researchers allowed scientists to accurately measure how the atmosphere’s composition and temperature changes. In 1902, Ernest Rutherford and Frederick Soddy’s radiation law led to the accurate dating of the Earth. This technique and its evolution resolved the long-standing controversy about the age of the solar system and our planet. Through radiometric dating, we have a much better understanding of the timeline of Earth’s atmospheric and biological history, from the beginning of life 3.5-4 billion years ago to the Anthropocene.
The arrival of life on Earth, and thus photosynthesis, led to dramatic changes to atmospheric composition. For example, photosynthesis consumes carbon dioxide, CO2, producing energy and biomass, but also releases molecular oxygen, O2. This results in the formation of ozone, O3, which is the most important atmospheric trace gas, because it absorbs biologically damaging ultraviolet radiation and thereby creates the stratosphere. European and later American scientists led the advances in our measurement and understanding of the O3, in particular the consequences of the human release of ozone depleting substances of which the chlorofluorocarbons, CFCs, are of particular importance. For example, European researchers have been at the forefront of developing the ground-based measurements of stratospheric ozone and water vapour (e.g. Gordon Dobson and Alan Brewer), of explaining how ozone is produced, removed and distributed in the stratosphere and mesosphere (e.g. Sydney Chapman, David Bates, Marcel Nicolet, Paul Crutzen).
Before, proposing the Gaia hypothesis, James Lovelock discovered that the CFCs were very long lived in the troposphere. The Gaia hypothesis considers that living matter on Earth collectively defines and regulates the material conditions necessary for the continuance of life. The long tropospheric lifetime of CFCs led Mario Molina and Sherwood Roland to identify the threat that the decay of CFCs in the stratosphere posed globally to upper stratospheric O3. In 1985 Joe Farman, Brian Gardner and Jon Shanklin discovered that Antarctica was experiencing a springtime loss of O3 in the lower stratosphere, now known as the ‘ozone hole’. Many scientists in America, Europe and around the world then worked to explain the cause of this phenomenon. This physicochemical mechanism was previously unknown!
These discoveries generated public concern about the loss of the upper atmospheric ozone protective layer. This led to the UN Vienna Convention for the Protection of the Ozone Layer, the Montreal Protocol and its amendments. These actions have effectively banned the production of several ozone depleting substances. The parties to the Montreal protocol, together with the UN Environment Programme and the World Meteorological Organization, now monitor the human impact on atmospheric ozone through regular assessments, where European scientists have been very active.
Another area where Europe has contributed uniquely to the geosciences is the evolution of our understanding of air pollution. Robert Angus Smith coined the term ‘acid rain’ in 1872 and Henry Antoine Des Voeux was the first to use the term ‘smog’ in 1905 to describe the winter air conditions in London. This toxic brew results from the pollutants, released from fossil combustion used for domestic heating and industry. After the ‘Great Smog’ of 1952, the UK’s Clean Air Act was the first legislation to limit pollutant emissions from coal burning. Nevertheless, coal and oil combustion across northern Europe led to acid deposition in the lakes of Scandinavia. The resultant controversy was resolved in Europe by legislation, which introduced the scrubbing of sulfur dioxide, SO2, from coal-fired power station emissions in the late 1980s. Photochemical smog, first identified in Los Angeles in 1948, was observed in Europe from the 1970’s onwards. With industrialisation over the past 50 years, summer smog, which produces toxic aerosols, O3 and other trace gases, and winter smog and thus acid deposition, have become worldwide issues. Air quality is now recognised to be of key importance for human health. Research from European and American scientists and increasing public concern led to the UN Economic Commission for Europe Convention on Long-range Transboundary Air Pollution, which aims to reduce pollution.
The issue of man-made climate change in all its complexity has also become the major global public concern in the past 50 years, from the first accurate carbon dioxide measurements at the Mauna Loa Observatory to the recent Fridays from Future movement, which began in Sweden with protests from student climate activist Greta Thunberg. The concerns about man-made climate change led to the establishment of the Intergovernmental Panel on Climate Change (IPCC) in 1988 to provide policymakers with regular scientific assessments on the current state of knowledge about climate change. The first chair of IPCC working group 1 was John Houghton. Many European scientists have subsequently played leading roles and contributed to IPCC and the United Nations Framework Convention on Climate Change (UNFCCC), which was adopted to provide international environmental policy to address climate change.
John Houghton was also a European pioneer in the field of remote sensing of atmospheric constituents and parameters from space. While NASA and in the Soviet Union spearheaded many developments in Earth observation, Europe’s contribution began with predecessors of what became the European Space Agency and its development of MeteoSat and the European Research Satellites: ERS-1, ERS-2 and Envisat. European scientists became leaders in the spaced based global measurement of tropospheric constituents, sea surface temperature, and the cryosphere by developing and exploiting instrumentation on these pioneering and subsequent Earth observation missions.
Which European researcher from the last century has influenced your work the most?
I am indebted to my teachers, research supervisors and scientific mentors (in particular Brian Thrush, Richard Wayne, Geert Moortgat and Dieter Perner), who all have influenced my research. I am also grateful to my many colleagues, students and scientific friends, who have shared my journey in research.
However, the person, who most influenced my work, and with whom I had the good fortune to undertake research for and with is atmospheric chemist Paul J. Crutzen. He is a brilliant ideas man, and in part a Descartes-like philosopher. He has made huge contributions to our understanding of the physics and chemistry of the atmosphere, especially stratospheric and tropospheric ozone, biomass burning, nuclear winter and climate change. He has a ‘why not’ rather than a ‘why’ approach to tackling new and challenging scientific issues. He recognised that pollution from the production of energy and food needed to sustain the growing population and provide its high standard of living was a primary cause for the depletion of stratospheric ozone, air pollution and recent warming of planet Earth. By proposing that, because of this, the Earth has entered a new geological epoch the Anthropocene, he has provided a phenomenological approach, which unites the scientific communities researching human impact on the environment with those tasked to develop policies to achieve sustainable development.
For the next generation of researchers, what skills/technology/concepts do you think will be the most important for advancing the next century of geoscience?
The Earth has never previously been in its current and evolving condition. We are data sparse with respect to the challenge of understanding, predicting and mitigating the changing regional impacts, which will occur in the evolving Anthropocene. Consequently the key cognitive and technical skills required for the next generation of scientists to advance the geosciences address the need to evolve, extend and enhance how we measure and interpret the changes in trace constituents and parameters of the Earth system. These measurements are essential to provide an early warning of change and its consequences, as the state of the Earth system responds to human activity or natural phenomena. Consequently, I recommend that student obtain skills in:
- the physical basis of ‘state of the art’ detection techniques, and
- the exploitation of the incredible advancement in computational power and intelligence.
The above requires a synergetic approach, multidisciplinary and possibly transdisciplinary research, based on sound disciplinary knowledge.
What do you think will be the biggest challenges for the geosciences in the next century?
The history of discoveries in the geosciences is a fascinating story, involving unexpected and perplexing observations, followed by experimentation, theory, and modelling of the unexplained phenomena to identify their physicochemical, geologic, biogeochemical or anthropogenic origins.
The Earth is in uncharted territory, since it entered the Anthropocene. Thus, one huge challenge is to provide in a timely fashion an adequate ‘fit for purpose’ global observing system. Similarly, to understand, mitigate and adapt to change, we must advance our understanding of the geosciences by studying the physical, chemical and biological processes or mechanisms, which determine the behaviour of the Earth system from the local to the global scale, and thereby improve the accuracy and predictive capability of models. The timely provision of accurate knowledge about the Earth and its atmosphere during the evolution of the Anthropocene is an essential prerequisite to limit the human impact on the Earth and thereby achieve sustainable development in the next century.
Interview by Olivia Trani, EGU Communications Officer
You can find more of our interviews on a century of geoscience in Europe here.