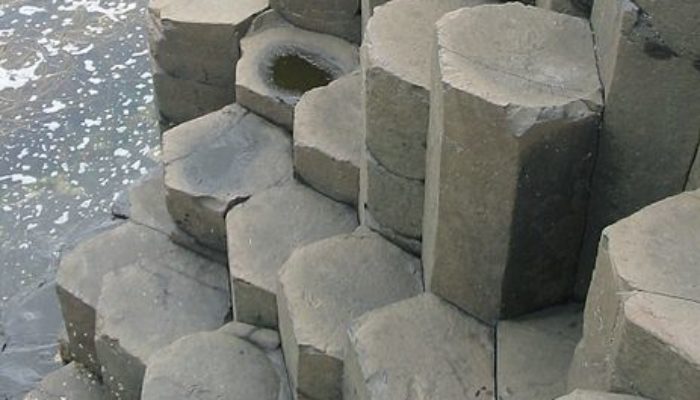
What does an insect eye, Saturn’s North pole, beeswax, and a rock formation called columnar basalts all have in common? They are all hexagonal in shape. Why is this the case?
Hexagons are the most efficient way to fill a space with the least amount of material
Figure 1. A circle in 2D and a sphere in 3D are the shapes that enclose the maximum volume possible with the least surface area. This allows to minimize external forces, such as surface tension, and it is for this reason that bubbles are round . When two circular objects interact, they form a straight line at their intersection (a plane in 3D), minimizing the surface area at their contact.If the interaction involves three or more circles, the minimization of the surface produces triple junctions at 120 degrees, forming the vertices of the hexagonal shape we see so much in nature.
The science of bubbles and bubble sculptures is very interesting and I do encourage you to go check out some other blogs that talk about bubble geometry in more detail (Fig. 1), however lets now talk about hexagonal basalts.
Columnar Basalts form by cracking when igneous rock rapidly cools after being emplaced. They constitute some of the most unique and awe-inspiring geological features we see on Earth. They are so regular and geometrically organised they look hand cut or man made. Some stories in mythology hypothesize they were built by giants (check our previous post about the Giant’s Causeway myth), or by the Great Spirit (wait for the next post about the Devils Tower myth). Once you analyse the physics behind them though, it becomes easier to understand why columnar basalt organise into such perfect geometrical shapes.
Imagine a big pool of lava (molten basaltic rock) that has settled and isn’t being topped up by more lava. Just like hot stew, the lava will cool faster at the edges and on the top, the solidified lava takes up less space than the molten lava because it contracts as it cools. The cooler contracted rock pulls on the still molten lava in the middle of the pool to the edge evenly. This generates cracks in the lava as the whole surface cools, much like dried mud (or dried starch slurry! Fig. 2a).
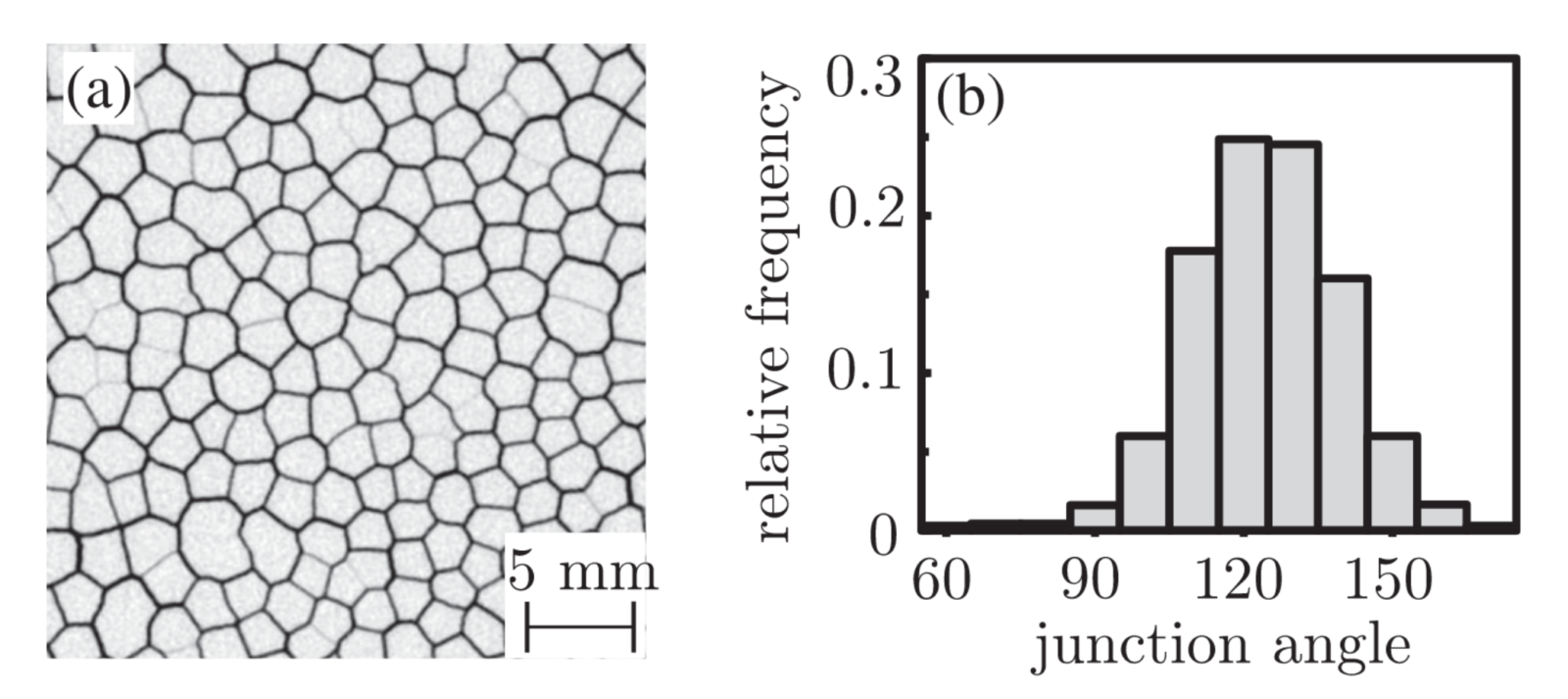
Figure 2. From Hofmann et al., (2015) (a) 2D slice extracted from a 3D tomogram of dried starch slurry columns due to shrinkage crack propagation, showing a tendency towards regular hexagons. (b) Relative frequency of angles in convex polygons of the column cross sections analysed in the tomogram.
If the lava is well mixed and smooth it will crack evenly and in predictable and repeated patterns; the mechanics of this are shown in Figure 3. during the cooling, the produced tensile stress accumulates elastically and initially generates randomly distributed micro-fractures while the cooler parts of the lava are pulling on other parts. Such micro-fractures subsequently tend to develop into a more organized set of polygonal fractures (Lamur et al., 2018); the most efficient regular pattern to relieve the most tension on the rock is if it cracks into regular hexagonal shapes.
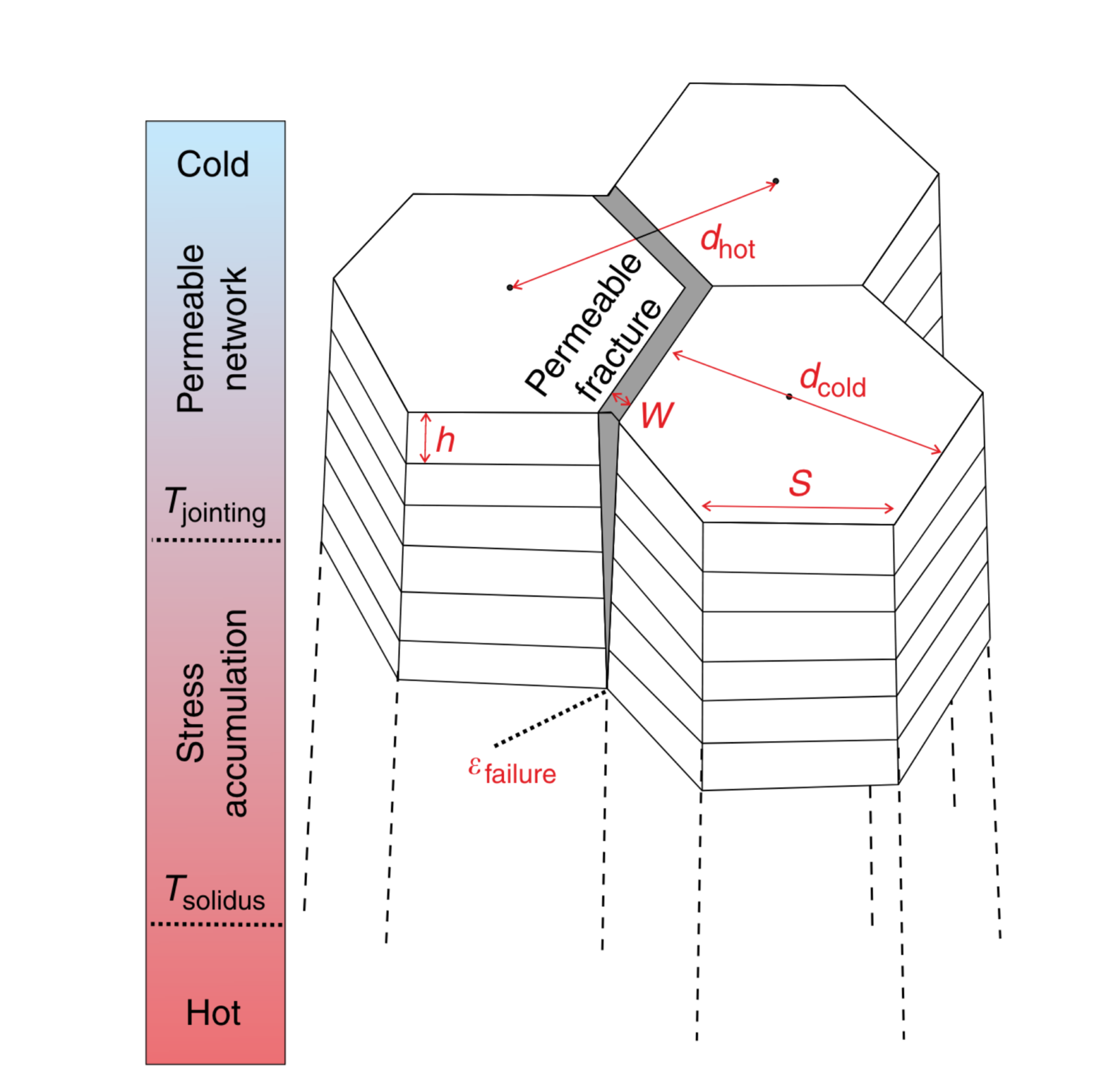
Figure 3. From Lamur et al., (2018) Fig. 1 Columnar jointing model. Tensile fractures between basalt columns form due to accumulation of tensional strain induced by thermal contraction between two focal points (dhot). The crack forms where the resistance to failure of the rock (εfailure) is exceeded.
At first the hexagonal shapes only exist on the surface of the lava but the hexagonal surface cracks propagate downwards into the lava as the deeper parts of the lava, initially kept molten by insulation, eventually cool too. Much the same as splitting wood, the surface cracks are weak points which propagate deep into the rock. This is how meter tall columnar basalts form.
Sometimes the presence of impurities in the lava will affect the way it cools down, causing some columns to develop 5 (pentagon) or 7 (heptagon) sides but the pattern “nature favours” (i.e. the pattern that is most efficient) is hexagons. The same pattern can be replicated at home with a starch slurry, like as Hofmann et al., (2015) did in a lab. Hofmann et al. (2015) looked inside of the dried starch using tomography, and measured the angles that form between the polygons as the slurry dries. Nature wants the 120 degree angle of a hexagon and 120 degrees is the most frequently occurring angle (Fig. 2b); but of course the model isn’t perfect and local effects will reduce or increase the angle – making a pentagon, heptagon or another irregular shape. In the case of the slurry, this may be because the slurry dried unevenly (i.e., if there was a temperature difference or a breeze) or if the slurry wasn’t mixed well enough and parts were stickier or runnier than others, or also if there was some contaminant in the slurry like leftover sugar. All the same can be applied to lava. Many columnar basalts do have imperfections, most likely because of one or more of the reasons mentioned above.
How far down do the columns go?
Columns (or colonnade) can become few meters to several tens of meters long (Fig. 4), however they can only continue to form to the base of the lava flow that was cooling.
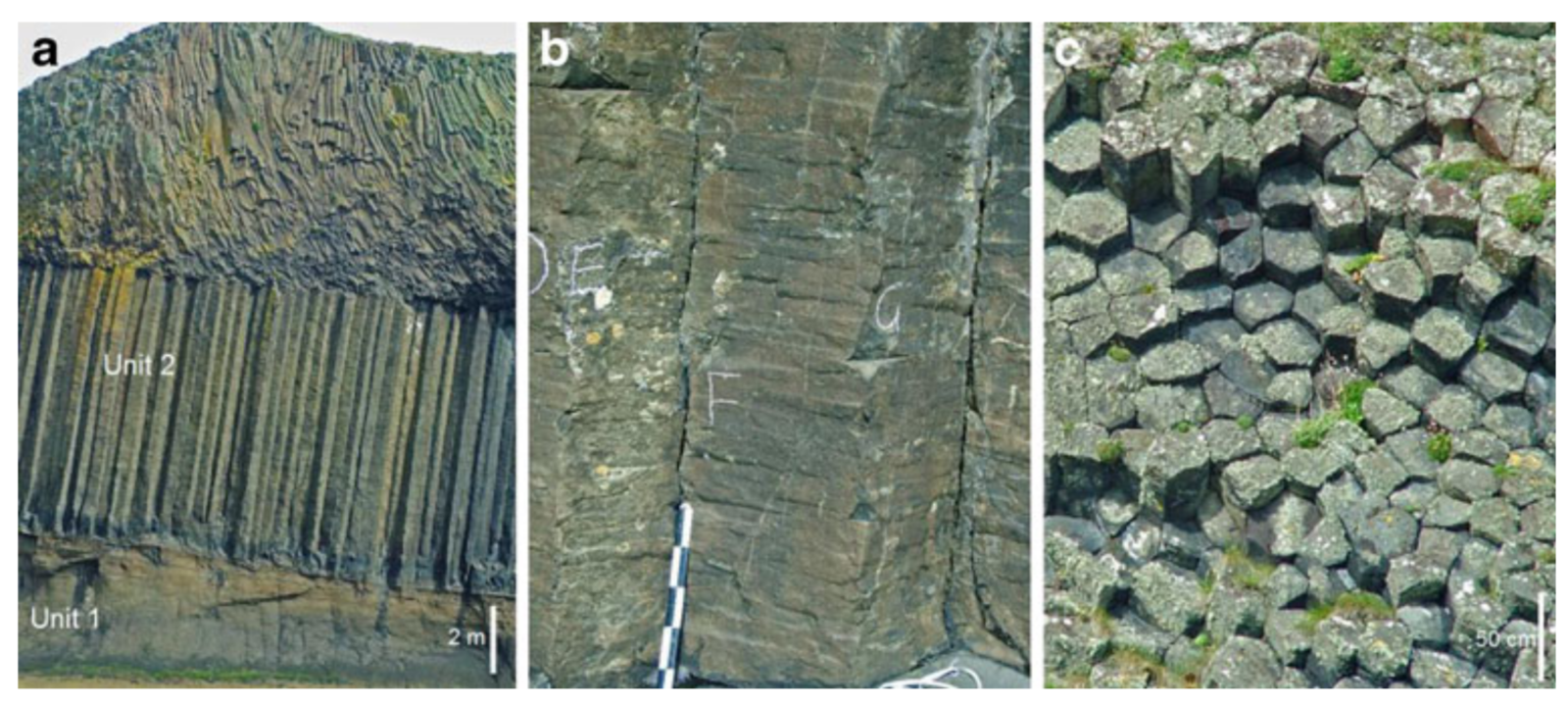
Figure 4. From Phillips et al., (2013) Field examples of jointing from Staffa. a) The Fingal’s Cave flow at its thickest point showing upper entablature, lower colonnade and underlying ignimbrite (a volcanic rock produced by explosive eruptions). b) Typical column faces with subhorizontal striae (each increment on the scale bar represents 10 cm). c) View of column tops in the entablature, Staffa, showing 4- to 6-sided, polygonal columns.
Subsequent lava flows, or parts of the lava flow above or below the columnar basalt formation can produce more fractured lava formations called entablature, which are formed by curved and irregular columns (Fig. 5). Different structures and geometries in lava generally occur because of the different chemical composition of different lava flows or the eventual interaction with water, which cause it to cool faster and asymmetrically. Although columnar jointing is typical of basaltic rocks, the same joint pattern can be also found on other types of volcanic rocks such as Phonolites (Zavada et al., 2015) and ignimbrites (Giordano and Cas, 2021).
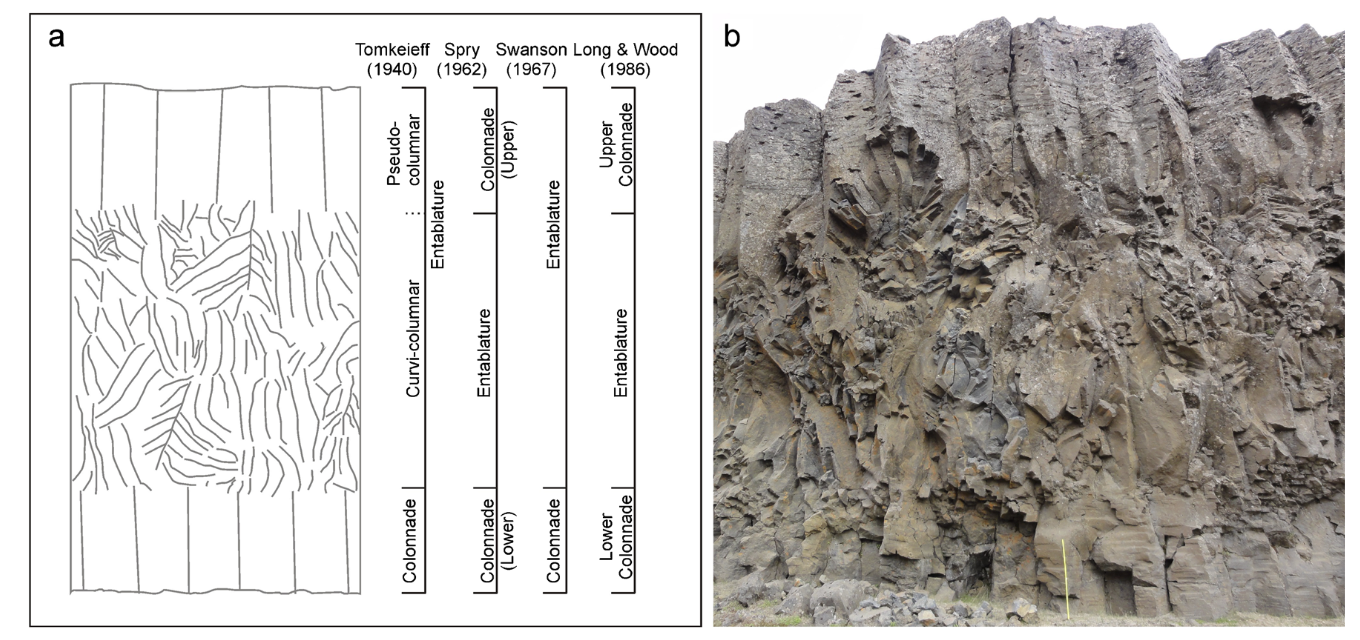
Figure 5. From Forbes et al., (2014), Entablature structure and nomenclature. a) Entablature sketch diagram. The nomenclature of Spry (1962) and Long and Wood(1986) was used to describe the outcrop. b) Section through the Búrfell lava near Gjáin with vesicular upper colonnade, a dominant entablature tier and a lower colonnade (base notexposed). Yellow metre rule for scale, flow is ~8 m thick.
Written by Hannah Davies and edited by Filippo Carboni and Samuele Papeschi
Jeroen Visser
Dear Hannah,
This is a part of the story.
Like clay , first forms a pattern with mainly 90 junction angles. After many iterations (drying /wetting ) cracks change place to form a 120 pattern.
Shrinking rock like basalt has no iterations, it has the striae.
If you look at the first meter at the base of the collonade at staffa. You see irregular colums because the cracking there started at a 90 pattern.
Lots of papers on this subject are found here :
http://www.lgoehring.com/Papers.html
A compact youtube lecture is found here :
https://www.youtube.com/watch?v=c3TpGtUZEjc ( Tuzo Wilson Lecture: Cracking the Giant’s Causeway with a Tabletop Experiment )
regards ,
Jeroen