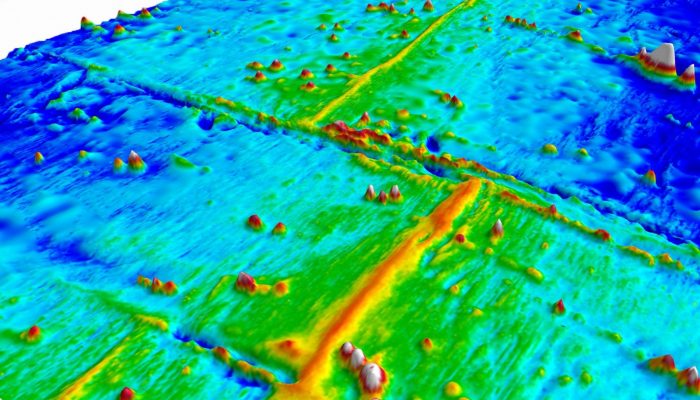
In this edition of Minds over Methods, Aurore Sibrant, postdoc at Bretagne Occidentale University (France) explains how she studies the shape of oceanic ridges, and which parameters are thought to control this shape. By using laboratory experiments combined with observations from nature, she gives new insights into how spreading rates and lithosphere thickness influence the development of oceanic ridges.
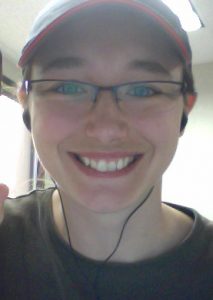
Credit: Aurore Sibrant
What controls the shape of oceanic ridges? Constraints from analogue experiments
Aurore Sibrant, Post-doctoral fellow at Laboratoire Géosciences Océans, Bretagne Occidentale University, France
Mid-oceanic ridges with a total length > 70 000 km, are the locus of the most active and voluminous magmatic activity on Earth. This magmatism directly results from the passive upwelling of the mantle and decompression melting as plates separate along the ridge axis. Plate separation is taken up primarily by magmatic accretion (formation of the oceanic crust), but also by tectonic extension of the lithosphere near the mid-ocean ridge, which modifies the structure of the crust and morphology of the seafloor (Buck et al., 2005). Therefore, the morphology of the ridge is not continuous but dissected by a series of large transform faults (> 100 km) as well as smaller transform faults, overlapping spreading centres and non-transform offsets (Fig. 1). Altogether, those discontinuities form the global shape of mid-ocean ridges. While we understand many of the basic principles that govern ridges, we still lack a general framework for the governing parameters that control segmentation across all spreading rates and induce the global shape of ridges.
Geophysical (Schouten et al., 1985; Phipps Morgan and Chen, 1993; Carbotte and Macdonald, 1994) and model observations (Oldenburg and Brune, 1975, Dauteuil et al., 2002, Püthe and Gerya, 2014) suggest that segmentation of oceanic ridges reflects the effect of spreading rate on the mechanical properties and thermal structure of the lithosphere and on the melt supply to the ridge axis. To understand the conditions that control the large-scale shape of mid-ocean ridges, we perform laboratory experiments. By applying analogue results to observations made on Earth, we obtain new insight into the role of spreading velocity and the mechanical structure of the lithosphere on the shape of oceanic ridges.
Laboratory experiments
The analogue experiment is a lab-scale, simplified reproduction of mid-oceanic ridges system. Our set-up yields a tank filled from bottom to top by a viscous fluid (analogous to the asthenosphere) overlain by the experimental “lithosphere” that can adopt various rheologies and a thin surface layer of salted water. This analogue lithosphere is obtained using a suspension of silica nanoparticles which in contact with the salted water emplaced on the surface of the fluid causes formation of a skin or “plate” that grows by diffusion. This process is analogous to the formation of the oceanic lithosphere by cooling (Turcotte and Schubert, 1982). With increasing salinity, the rheology of the skin evolves from viscous to elastic and brittle behaviour (Di Giuseppe et al., 2012; Sibrant and Pauchard, 2016).
The plate is attached to two Plexiglas plates moving perpendicularly apart at a constant velocity. The applied extension nucleates fractures, which rapidly propagate and form a spreading axis. Underlying, less dense, fresh fluid responds by rising along the spreading axis, forming a new skin when it comes into contact with the saline solution. By separately changing the surface water salinity and the velocity of the plate separation, we independently examine the role of spreading velocity and axial lithosphere thickness on the evolution of the experimental ridges.
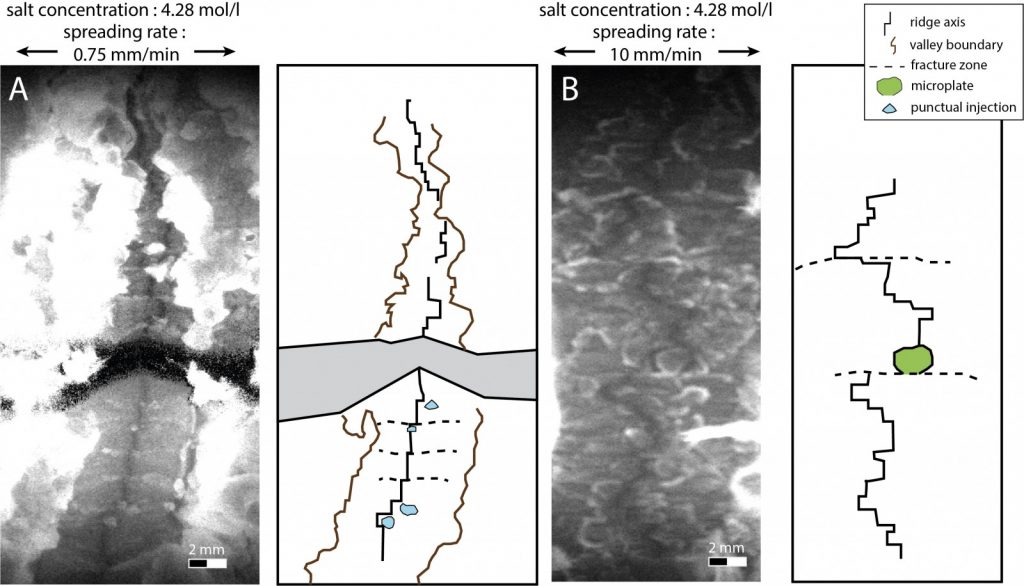
Figure 2. Close up observations of analogue mid-oceanic ridges and schematic interpretation for different spreading velocity. The grey region is a laser profile projected on the surface of the lithosphere: the laser remains straight as long as the surface is flat. Here, the large deviation from the left to centre of the image reveals the valley morphology of the axis. Credit: Aurore Sibrant.
Analogue mid-oceanic ridges
Over a large range of spreading rates and salinities (Sibrant et al., 2018), the morphology of the axis is different in shape. The ridge begins with a straight axis (initial condition). Then during the experiment, mechanical instabilities such as non-transform offset, overlapping spreading centres and transform faults develop (Fig. 2) and cause the spreading axis to have a non-linear geometry (Fig. 3). A key observation is the variation of the shape of the analogue ridges with the spreading rate and salinities. For similar salinity and relative slow spreading rates, each segment is offset by transform faults shaping a large tortuous ridge (i.e. non-linear geometry). In contrast, at a faster spreading rate, the ridge axis is still offset by mechanical instabilities but remains approximately linear.
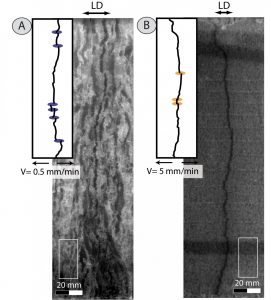
Figure 3. Ridge axis morphology observed in the experiments and schematic structural interpretations of the ridge axis, transform faults (orange ellipsoids) and non-transform faults (purple ellipsoids). Measurements of lateral deviation (LD) correspond to the length of the arrows. For comparison, white squares represent the size of closeup shows in Fig 2. Credit: Aurore Sibrant.
We can quantify the ridge shape by measuring the total lateral deviation, which is the total accumulated offset of the axis, when the tortuosity amplitude becomes stable. For cases with similar salinities, the results indicate two trends. First, the lateral deviation is high at slow spreading ridges and decreases within increasing spreading rate until reaching a minimum lateral deviation value for a given critical spreading rate (Fig 4A). Then the lateral deviation remains constant despite the increasing spreading rate. Experiments with different salinities also present a transition between tortuous and linear ridges. These two trends reflect how the lithosphere deforms and fails. In the first regime, the axial lithosphere is thick and is predominantly elastic-brittle. In such cases, the plate failures occur from the surface downwards through the development of faults: it is a fault-dominated regime. In contrast, for faster spreading rate or smaller salinities, the axial lithosphere is thin and is predominantly plastic. Laboratory inspection indicates that fractures in plastic material develop from the base of the lithosphere upwards: it is a fluid-intrusion dominated regime.
Comparison with natural mid-oceanic ridge
In order to have a complete understanding of the mid-oceanic ridge system, it is essential to compare the laboratory results with natural examples. Hence, we measure the lateral deviation of nature oceanic ridges along the Atlantic, Pacific and Indian ridges. The measurements reveal the same two regimes as found in laboratory data. The remaining step consists of finding the appropriate scaling laws to superpose the natural and experiment data. This exercise requires dynamics similarity between analogue model and real-world phenomena which is demonstrated using dimensionless numbers (Sibrant et al., 2018). Particularly, the “axial failure parameter – πF” describes the predominant mechanical behaviour of the lithosphere relative to its thickness. Low-πF accretion is dominated by fractures in a predominantly elastic-brittle lithosphere: the lateral deviation of the ridges is tortuous, while at higher pF, accretion is dominated by intrusion in a predominantly plastic lithosphere: the shape of the mid oceanic ridges is mostly linear (Fig 4B).
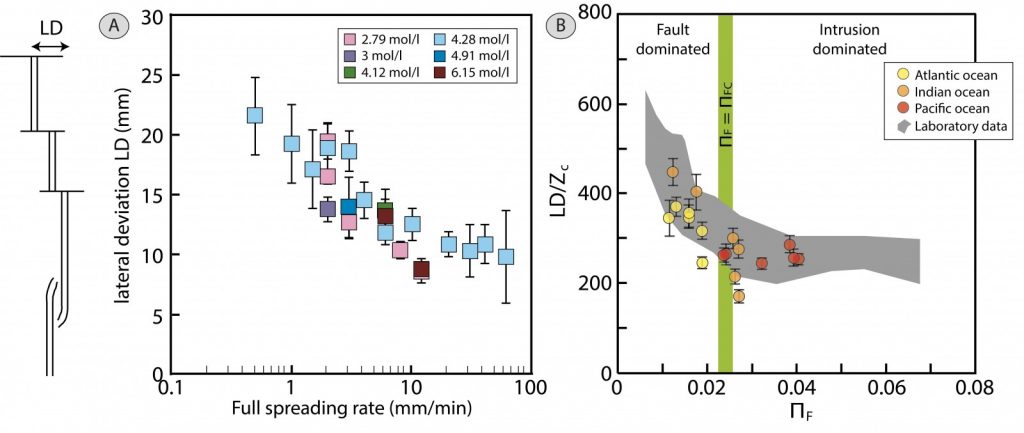
Figure 4. (A) Lateral deviation values measured in the experiments in function of the spreading rate velocities and salinities. (B) Evolution of the lateral deviation of the ridge axis, normalized by the critical axial thickness (Zc) relative to the axial failure parameter. Dark grey is the laboratory experiments and the colored circles are the Earth data. Adapted from Sibrant et al., 2018.
Our experiments give insight into the role of axial failure mode (fault-dominated or intrusion-dominated) on the shape of mid-oceanic ridges. In the future, we want to use this experimental approach to investigate the origin of mechanical instabilities, such as transform faults or overlapping spreading centres. This experimental development and results are a collaborative work between Laboratoire FAST at Université Paris-Saclay and Department of Geological Sciences at the University of Idaho and involves E. Mittelstaedt, A. Davaille, L. Pauchard, A. Aubertin, L. Auffray and R. Pidoux.
References
Buck, W.R., Lavier, L.L., Poliakov, A.N.B., 2005. Modes of faulting at mid-ocean ridges. Nature 434, 719-723.
Schouten, H., Klitgord, K.D., Whitehead, J.A., 1985. Segmentation of mid-ocean ridges. Nature 317, 225-229.
Carbotte, S.M., Macdonald, K. C., 1994. Comparison of seafloor tectonic fabric at intermediate, fast, and super fast spreading ridges: Influence of spreading rate, plate motions, and ridge segmentation on fault patterns. J. Geophys. Res. 99, 13609-13631.
Phipps Morgan, J., Chen, J., 1993. Dependence of ridge-axis morphology on magma supply and spreading rate. Nature 364, 706-708.
Oldenburg, D.W., Brune, J.N., 1975. An explanation for the orthogonality of ocean ridges and transform faults. J. Geophys. Res. 80, 2575-2585.
Dauteuil, O., Bourgeois, O., Mauduit, T., 2002. Lithosphere strength controls oceanic transform zone structure: insights from analogue models. Geophys. J. Int. 150, 706-714.
Püthe, C., Gerya, T., 2014. Dependence of mid-ocean ridge morphology on spreading rate in numerical 3-D models. Gondwana Res. 25, 270-283.
Turcotte, D., Schubert, G., Geodynamics (Cambridge Univ. Press, New York, 1982).
Di Giuseppe, E., Davaille, A., Mittelstaedt, E., Francois, M., 2012. Rheological and mechanical properties of silica colloids: from Newtonian liquid to brittle behavior. Rheologica Acta 51, 451-465.
Sibrant, A.L.R., Pauchard, L., 2016. Effect of the particle interactions on the structuration and mechanical strength of particulate materials. European Physics Lett., 116, 4, 10.1209/0295-5075/116/49002.
Sibrant, A.L.R., Mittelstaedt, E., Davaille, A., Pauchard, L., Aubertin, A., Auffray, L., Pidoux, R., 2018. Accretion mode of oceanic ridges governed by axial mechanical strength. Nature Geoscience 11, 274-279.