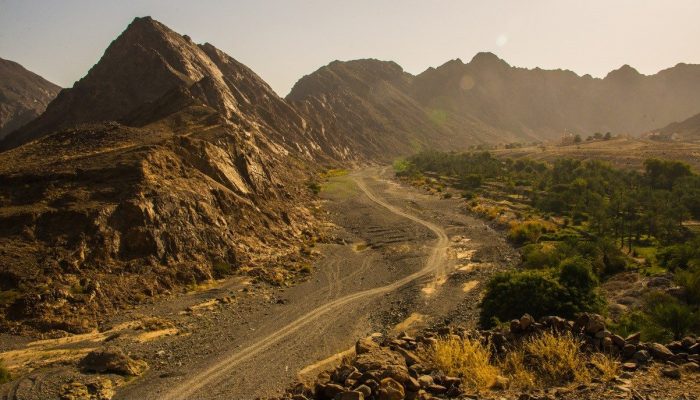
For this first Minds over Methods of 2019, we invited Christopher Spencer, Senior Research Fellow at Curtin University in Australia, to tell us something about tectonochemistry. By applying geochemistry to tectonic processes, it is possible to get more insight into the different stages of the rock cycle. By combining fieldwork and geochemical analyses of the Oman/UAE ophiolite, Chris and his co-workers believe they found the first direct and in-situ evidence of sediment melting in the mantle.
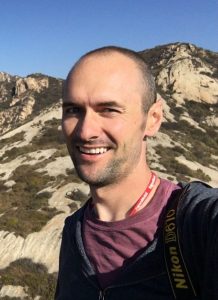
Credit: Christopher Spencer
Tectonochemistry of Melting Mud in the Mantle, evidence from the Oman/UAE ophiolite
Christopher Spencer, Senior Research Fellow, Curtin University, Australia
The rock cycle is the first thing we learn in Geology 101. Magma and lava cool to form igneous rocks. Igneous rocks then erode to form sediment, which forms sedimentary rocks as it is compacted. Increasing pressure and heat then create metamorphic rocks, which eventually will melt. In each of the transitions described in the rock cycle, tectonics is usually involved. Granite batholiths form in subduction zones and are uplifted and eroded in collision zones. The sediments derived therefrom are deposited along continental margins that are often then returned to subduction zones where they contribute to new magmatic systems. There is a wide array of tools that we can use to evaluate the role of tectonics in the rock cycle, of which geochemistry is able to provide insight into each stage of the process.
Applying geochemistry to tectonics is (unsurprisingly) referred to as tectonochemistry. Similar to tectonophysics, where geophysics is applied to address large-scale tectonic questions, tectonochemistry provides a unique view into geochemical proxies of tectonic processes. The melting of sediment along convergent margins is a classic tectonochemical problem, as the unique chemical signature of sediment found in a granite provides unequivocal evidence for the melting of a sedimentary rock. In collisional systems, like the Himalaya, tectonochemistry has been used to constrain the melting of meta-sedimentary rocks as crustal thickening and decompression drives dehydration of micas which leads to melting. Collisional systems provide clear and in situ evidence for sediment melting.
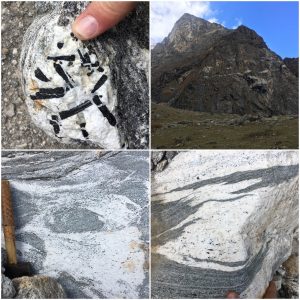
Figure 1: Clockwise from top left: tourmaline-bearing leucogranite from the Himalaya in NW India, leucogranite dykes intruding meta-sedimentary rocks exposed at 5000m altitude, in situ melting of meta-pelite and formation of leucogranite, incongruent melting of muscovite + plagioclase + quartz to form leucogranite but leaving the biotite behind. Credit: Christopher Spencer.
Sediments are also thought to melt in subduction systems, but given the difficulty of accessing the asthenosphere directly, it is more challenging to constrain the processes occurring deep in a subduction zone. The incorporation of sediment in subduction zones is often constrained using the geochemistry of the resulting magmatic rocks. The chemical signature of sediment provides a clear indication of its incorporation in the magma, but it is often unclear whether the contamination is occurring in the asthenospheric wedge or in the upper crust. For example, many granite batholiths contain zircon grains that are foreign to the host magma and whose age spectra match the detrital zircon age spectra of the adjacent sedimentary units. This relationship is a clear indication that sedimentary contamination occurred in the upper crust. Unfortunately, the geochemical proxies used to establish the sedimentary contamination only provide indirect evidence for the subduction of sedimentary material into the asthenospheric wedge. Such indirect evidence includes seismic stratigraphy showing sedimentary units being subducted beneath the forearc and whiffs of sedimentary geochemical signals in arc volcanics. Although these evidences point towards sediment being subducted deep into the asthenospheric wedge where it melts and contaminates the magmas coming off the subducting slab, they do not preserve direct evidence of sediment melting in the mantle.
To acquire direct evidence of processes happening deep in the mantle, I set my sights on the Oman/UAE ophiolite, where a thick succession of mantle peridotite is preserved beneath a complete stratigraphic section of oceanic crust. Previous work has shown that this ophiolite not only preserves an intact record of oceanic crustal stratigraphy, but also geochemical features of a subduction zone in the oceanic crust. This implies the ophiolite formed in a supra-subduction setting, where during the earliest phase of subduction, extension in the upper plate caused rifting and formation of oceanic crust above a subduction zone.
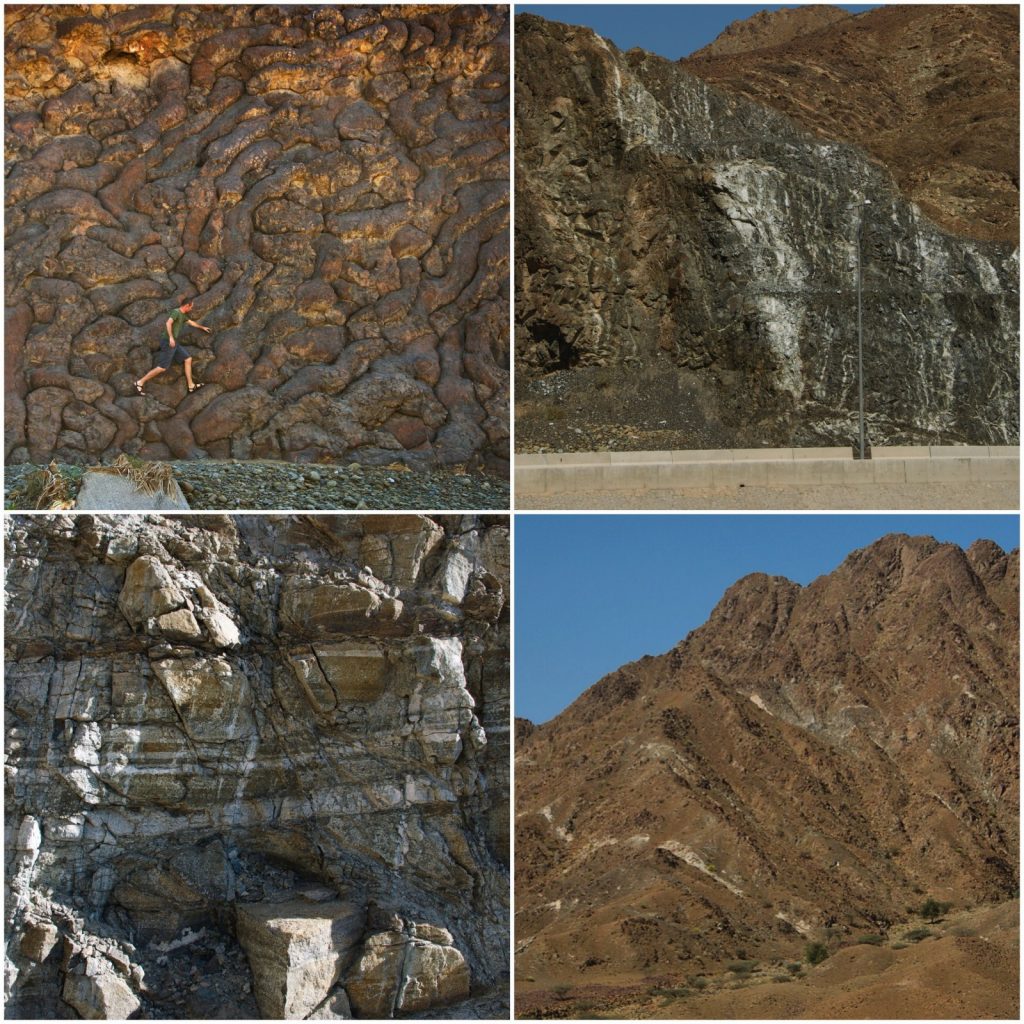
Figure 2: Oceanic crustal stratigraphy of the Oman/UAE ophiolite comprised of (clockwise from top left): pillow basalts, sheeted dykes, layered gabbros, and mantle peridotite. Credit: Christopher Spencer.
During fieldwork in the ophiolite, while traversing the 8-15 km thickness of the mantle peridotite, I encountered a number of granitoid dykes that cross cut the peridotite, but do not cross the petrologic Moho. Many of these dykes contained tourmaline, muscovite, biotite, and even andalusite, minerals that would be expected from the melting of sedimentary material. Finding these minerals in the mantle indicates these grantoid dykes formed from the melting of sedimentary material and here they were within the mantle! Subsequent analysis of zircon grains from these granitoid dykes revealed the age of these dykes was equivalent to the age of the overlying ophiolite providing bullet-proof evidence that they intruded while the ophiolite was forming above a subduction zone. To provide the nail in the coffin for a sedimentary origin, I performed oxygen isotope analysis of the zircon and quartz. Sedimentary material has a distinct oxygen isotopic composition and igneous rocks that are thought to have experienced sediment contamination have δ18O values that lie along mixing lines between a sediment end member and the mantle. The oxygen isotopic analyses of the sub-Moho granitoids of the Oman/UAE ophiolite revealed the highest δ18O values ever measured in igneous rocks, providing unequivocal evidence that these granitoids represent pure sediment melts. In a paper published in Geology (Spencer et al., 2017), my coauthors and I argue these igneous rocks represent the first direct and in situ evidence of sediment melting in the mantle. Lucky for us, we have just scratched the surface of the exciting things left to learn about these fascinating granitoids and I look forward to the opportunity to return to the Oman/UAE ophiolite.
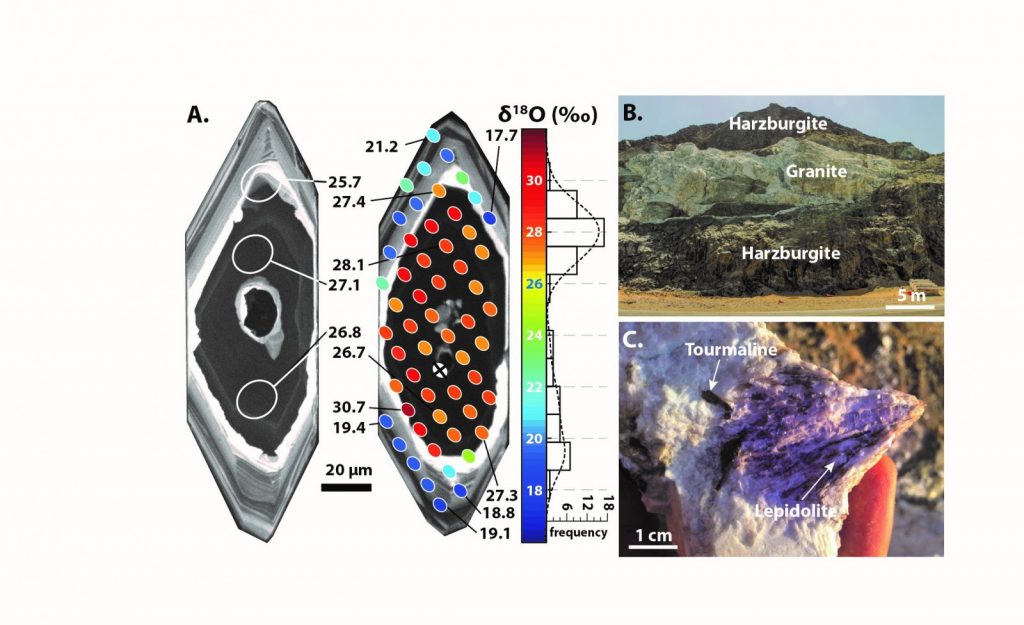
Figure 3: Sub-Moho granitoids of the Oman/UAE ophiolite: A) Cathodoluminescence image of a zircon shown with location and result of δ18O analyses. B) Photograph of sub-Moho granitoids. C) Hand sample of granite with tourmaline and lepidolite (lithium-bearing mica). Credit: Christopher Spencer.