June 2022: I was discussing the ongoing drought with my family over lunch, when my dad pointed to me and summarized things as follows: “You know, less snow in winter means less water in summer!” I almost choked … what? Not only was it the first time I realized my family had been listening to my scientific anecdotes for years, but I also had concrete evidence now that snow was entering public ...[Read More]
Why the 2022 Italian snow drought matters to you
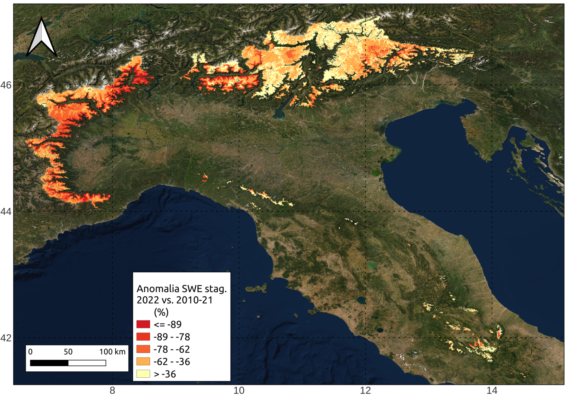