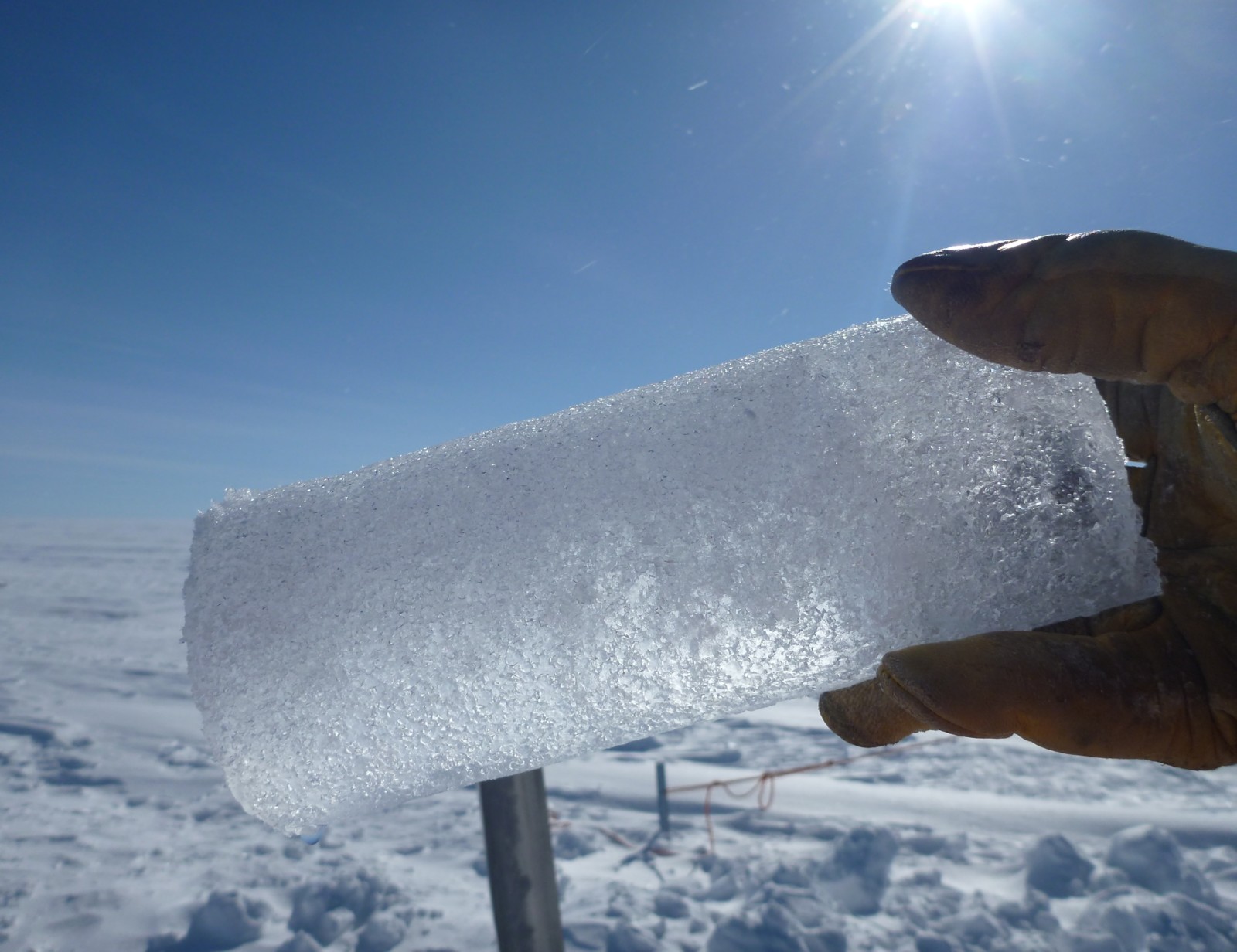
This part of a firn core was drilled from a firn aquifer. Notice that liquid water that was trapped within the firn is collecting on the left-hand side of the core [Credit: Ludovic Brucker, NASA Goddard Space Flight Center].
Hidden below the surface of some glaciers, liquid water can be found within what is called the firn layer – the upper layer of a glacier where snow compacts into glacier ice. Liquid water may persist there for up to many years, forming what scientists call “firn aquifers.” While observations of seasonal firn aquifers have existed since the mid to late 1900s in several mountain glaciers, recent studies discovered more significant and persistent firn aquifers along the perimeter of the Greenland Ice Sheet and even on an Antarctic ice shelf. Firn aquifers have piqued the interest of scientists because they:
- lubricate the glacier bed when they intermittently drain, therefore increasing the glacier’s speed,
- affect the process of snow compacting into glacier ice,
- may affect the temperature of the glacier,
- may contribute to fracturing and breakup of ice shelves, if located there.
In this blog post, you will find an overview of where firn aquifers have been found, how they form, and why they are a key component of the liquid water cycle of the ice sheets in the present and future.
Where do firn aquifers form?
WHERE IN THE WORLD?
Firn aquifers have been found across the world, including:
- Storglaciären, Sweden,
- South Cascade Glacier, USA,
- Holtedahlfonna Ice Field, Svalbard,
- Upper Seward Glacier, Canada,
- SE perimeter of the Greenland Ice Sheet (containing a significant 0.4 millimeters of global sea-level equivalent),
- SW and NW perimeter of the Greenland Ice Sheet (but a smaller extent than the SE), and
- within the Wilkins Ice Shelf, Antarctic Peninsula.
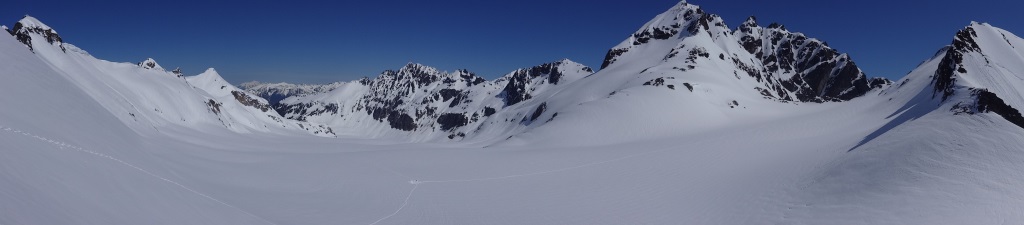
South Cascade Glacier, USA, is one mountain glacier that has been known to seasonally store liquid meltwater in its firn [Credit: USGS].
WHERE ON A GLACIER?
The upper layer of a glacier is composed of compacted snow called firn. Imagine that a glacier’s existence begins as a snowflake. Over time, many, many snowflakes collect over many years, snowfall exceeds snow melt, and eventually the snow compacts to form glacier ice under the weight of overlying snow and from other processes responding to climate and other stresses. Compaction occurs through time to continue to turn snow into glacier ice. Firn represents the intermediate stage between the transition from snow to glacier ice.
Like snow, firn consists of a mixture of both ice crystals and air pockets. Firn is denser (less air pockets) than freshly fallen snow but less dense (more air pockets) than glacier ice. In contrast to the glacier ice beneath, much of the firn layer is both porous and permeable – which means that air or water can be stored in and transmitted through this part of a glacier.
During summer on a glacier, snow or ice at its surface can melt from the heat of the sun and form liquid meltwater on the surface of the glacier. Where does this meltwater go? This is an important question that scientists want to know for understanding the mass balance of glaciers and their impact on sea-level rise. Mass balance is the net change of the glacier’s mass over some time period, and can be thought of as the glacier’s bank account, with losses (e.g., melting of snow or ice) and gains (e.g., snowfall). Mass loss such as meltwater from the glacier may ultimately end up in the oceans, but how this happens and when it happens is critical to understand.
In general, meltwater can either (1) evaporate, (2) refreeze into ice layers, (3) flow into crevasses (fractures in the glacier) or moulins (holes in the glacier), which funnel water to the bottom of the glacier, (4) directly run off into the ocean or adjacent glacial lakes, (5) form lakes on the surface of the glacier, and/or (6) percolate downward due to gravity into the firn layer, and then persist there – for solely the summer season, or through the winter season(s) for up to multi-year periods – to form a firn aquifer.
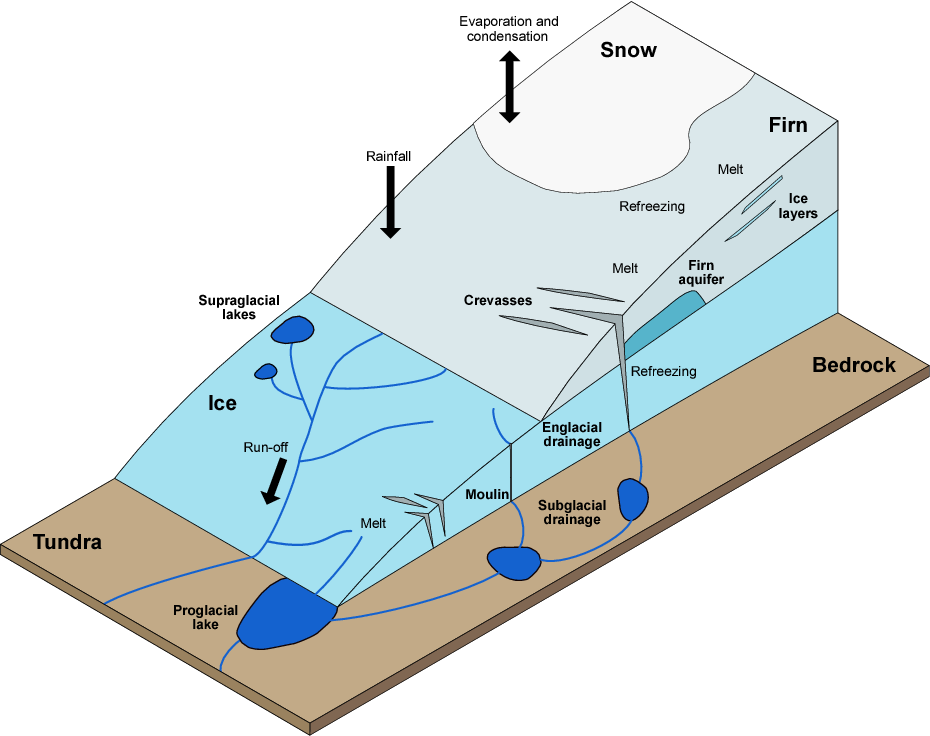
Many features and processes affect how liquid water moves through the Greenland Ice Sheet [Credit: Steger and others, 2017].
How do we measure firn aquifers?
While studying where meltwater goes in a glacier remains challenging, some scientists are exploring creative ways to examine the inner hidden workings of a glacier (i.e., for processes #3), where some of this meltwater goes. Since firn aquifers (from process #6) exist within the firn layer and are difficult to observe directly, you may be wondering how scientists examine them too. Some methods include:
- Ice-penetrating radar – This technique involves sending out radio waves into a glacier and measuring the time of the waves’ return after they are reflected at certain boundaries (e.g., layers within the glacier, the bedrock/glacier interface, the air/glacier interface, and the firn-aquifer water table).
- Firn cores and borehole studies – These techniques involve drilling into the upper part of the glacier and analyzing the extracted core of firn or putting instruments down inside the hole in the glacier to measure certain variables, such as temperature.
- Slug test – This test involves adding or removing water and monitoring the response of the aquifer.
- Dye tracer test – Releasing dye into the glacier provides information on water flow between the input and detection site.
- Computer modeling – Models use physics and mathematical relationships to simulate the complex real-world system.
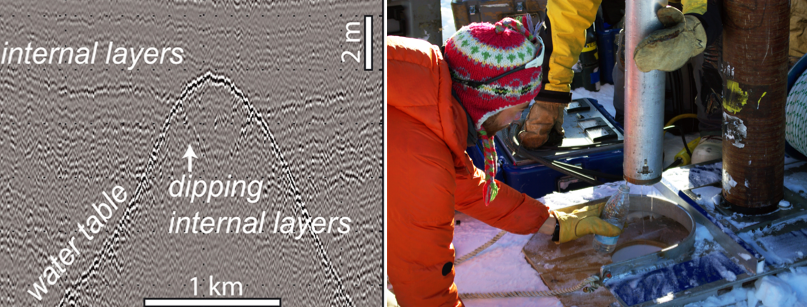
Two of the ways to study firn aquifers. Left: Ice-penetrating radar images can be used to “see” firn aquifers. The arching bright reflection is the upper surface (i.e. the “water table”) of the firn aquifer within southeast Greenland. The less bright reflections that trend horizontally throughout the image and dip near the aquifer are the internal layers of the glacier. [Credit: Adapted from Miège and others (2013)] Right: Scientists extract a firn core from a firn aquifer while collecting liquid water that is draining out from the core. [Credit: Ludovic Brucke, NASA]
How do perennial firn aquifers form?
Liquid meltwater within a cold glacier may sound physically impossible. However, it can exist through a balance between melting at the surface of the glacier, refreezing of the liquid meltwater, warming of the firn, and snowfall in the winter. Let’s specifically consider how this process happens with firn aquifers that persist through the winter, known as “perennial firn aquifers,” which impact the large ice sheets’ mass balance, runoff to the oceans, and thus contribution to sea-level rise.
Perennial firn aquifers form, generally, where there is:
- high summer surface melting,
- high snowfall, and
- moderate winter air temperatures.
In the winter, snow falls on the surface of the ice sheet, which will house pore space in the firn. Those pockets of air will later be needed to store meltwater in.
During summer, the warmth from the sun melts some of this snow, forming liquid meltwater on the surface of the glacier. This meltwater percolates down into and refreezes within the firn layer, which will warm the surrounding firn (the freezing process releases heat). When the firn layer reaches 0°C, the liquid meltwater can:
- move to other parts of the firn without refreezing,
- saturate the firn layer to form a firn aquifer if there is enough meltwater, or
- replenish an existing firn aquifer.
While it was thought that storage of liquid water could be long term within perennial firn aquifers, new studies in 2018 and 2020 suggest that the liquid water flows relatively quickly (i.e., less than 30 years, and on average 6.5 years) through the firn aquifer to then be released through exit locations in the glacier’s water system, such as crevasses.
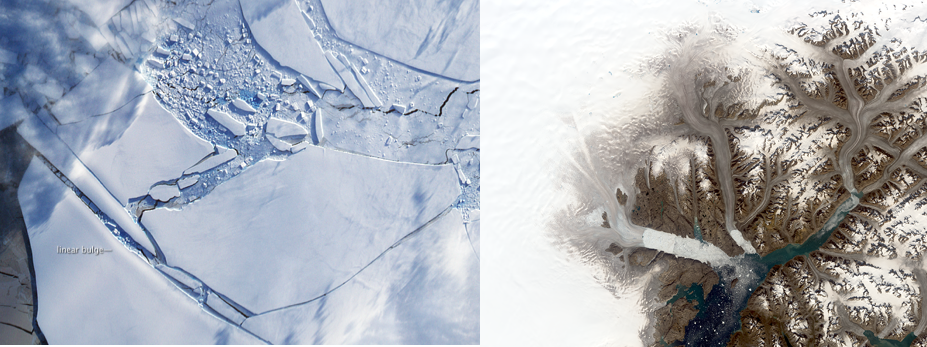
Firn aquifers have been observed within the Wilkins Ice Shelf, Antarctica (left) and Helheim Glacier, Greenland (right). They are expected to expand to other parts of the ice sheets that will become more temperate in the future [Credit (left to right): NASA/Jesse Allen, NASA/Christopher Shuman]
Summing up … the future and big picture
Firn aquifers in southeast Greenland, for example, are expected to expand due to global climate change in response to unprecedented melting on the ice sheet’s surface. New locations, such as glaciers on the Antarctic Peninsula or other parts of the Greenland Ice Sheet, may host firn aquifers in the future as they become more temperate. What does this mean for the glaciers and ice sheets? A more complete understanding of the implications of firn aquifers is needed, as these are not fully understood at present. But it is hypothesized that future impacts could include some of the following:
- increased lubrication of the glacier’s bed from the intermittent draining of firn aquifers, which would increase the glacier’s speed,
- increased influence on the temperature of the glacier,
- and contribution of firn aquifers to sea-level rise.
Firn aquifers will become even more critical in quantifying and predicting the mass balance of the ice sheets and their contribution to sea-level rise in the future.
Further Reading
- For a comprehensive description of the most well-studied firn aquifer in Greenland: Miller and others (2020) Hydrology of a perennial firn aquifer in Southeast Greenland: an overview driven by field data. Water Resources Research, 56 (8): e2019WR026348
- To learn more about how ice-penetrating radar can be applied to detect firn aquifers: Miège and others (2016) Spatial extent and temporal variability of Greenland firn aquifers detected by ground and airborne radars. Journal of Geophysical Research: Earth Surface, 121(12):2381-98
- For a description of the firn aquifer on Wilkins Ice Shelf, Antarctica: Montgomery and others (2020). Hydrologic properties of a highly permeable firn aquifer in the Wilkins Ice Shelf, Antarctica. Geophysical Research Letters, 47(22), e2020GL089552
- For information on water storage in South Cascade Glacier: Fountain (1989) The storage of water in, and hydraulic characteristics of, the firn of South Cascade Glacier, Washington State, USA. Annals of Glaciology. 13 (1989): 69-75.
- For information on the liquid water balance of the Greenland Ice Sheet: Steger and others (2017) The modelled liquid water balance of the Greenland Ice Sheet. Cryosphere. 11(6), 2507-26
Edited by T.J. Young
Annika Horlings is a PhD candidate at the Department of Earth and Space Sciences, University of Washington (UW), USA. Her primary research interests are in using modeling and ice-penetrating radar to study changes in near-surface processes of glaciers and ice sheets and their implications on ice-sheet mass balance. At the UW Program on Climate Change and the UW College of the Environment, she also avidly pursues cryospheric science communication and outreach and works to further diversity, equity, and inclusivity initiatives. Contact email: annikah2@uw.edu