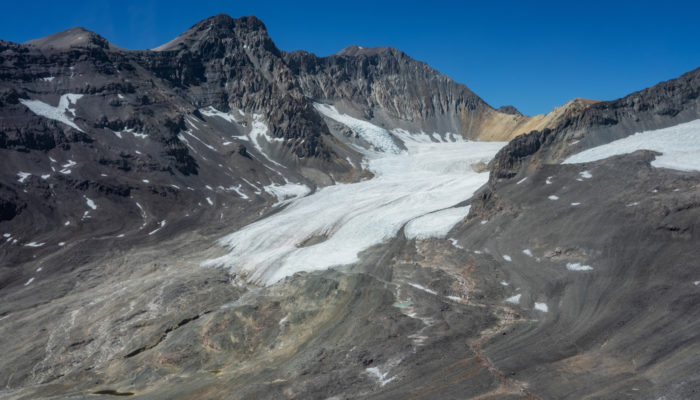
Central Chile has been facing a long dry period since 2010, marked by a high mean precipitation deficit, a so-called Mega Drought (MD) (Garreaud et al., 2019). This, besides long-term temperature increase (Burger et al., 2018; Falvey & Garreaud, 2009), has affected negatively the glaciers’ mass balance in the region mainly due to low snow accumulation throughout a hydrological year (which is from April to March).
Glaciers under pressure
The Olivares River basin (33º9’S – 70º11’W, 543 km²), a sub-basin within the Maipo River basin, is one of the most glaciated areas in Central Chilean Andes. It’s covered by ~80 km² (as measured in 2018) of different types of glaciers and cryospheric landforms (Figure 1). The main uncovered glaciers in the basin have experienced strong shrinkage and thinning in recent decades (Ayala et al., 2019; Farías-Barahona et al., 2020; Malmros et al., 2016), and because of it, some glaciers have been adding rocks and sediments to their surface, therefore changing their morphology into debris-covered glaciers or rock glaciers. A phenomenon that has been amplified by the unfavourable climate context.
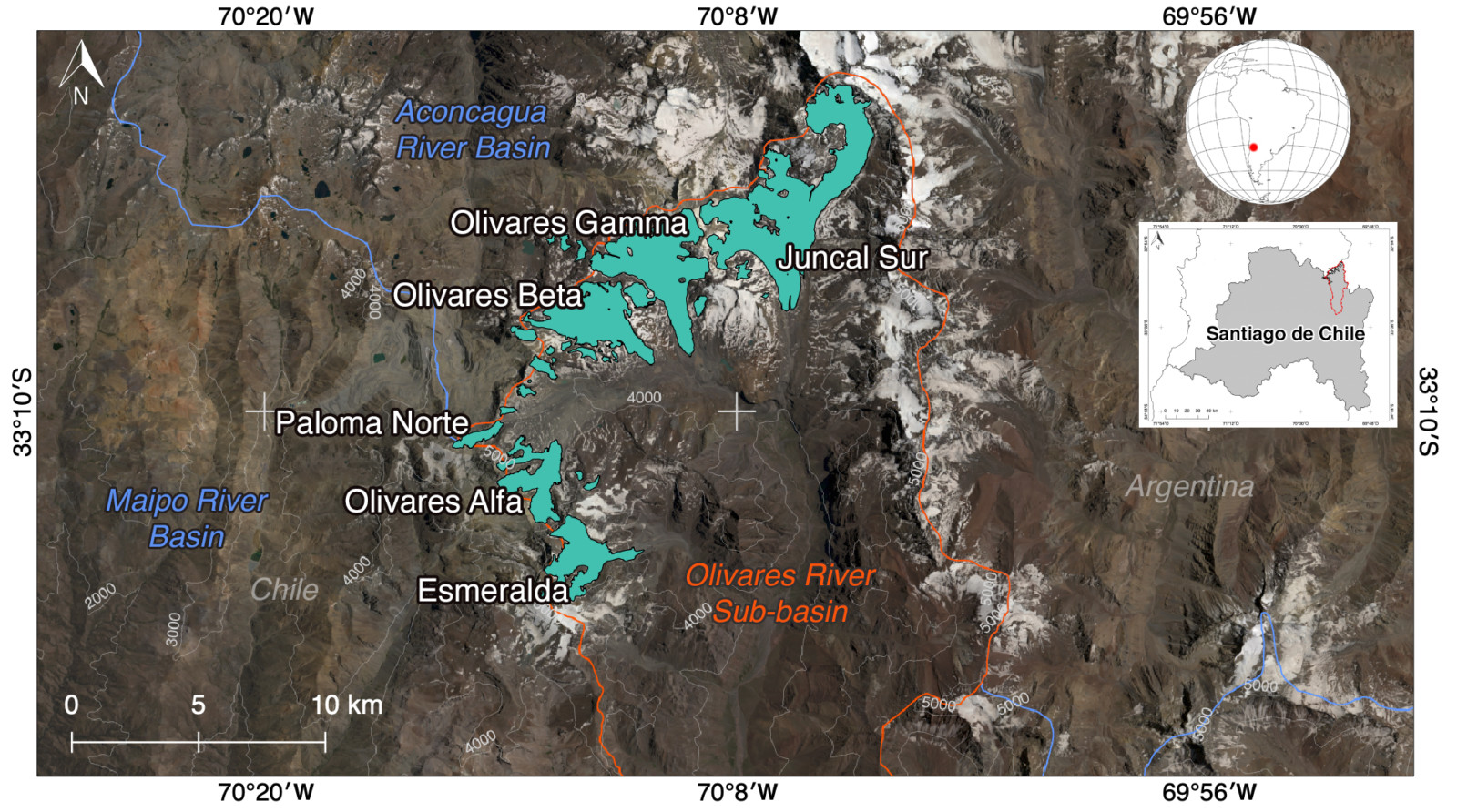
Figure 1. Location of the Olivares River sub-basin in Chilean Central Andes, the main glaciers are shown in light blue. Figure credit: CECs.
A glaciological monitoring program
Since 2012, we have carried out non-stop glaciological monitoring program in the upper Olivares River sub-basin (> 3000 m above sea level) in order to understand the local response of the glaciers to climate change and human activities. This program is focused on the monitoring of the main uncovered and debris-covered glaciers (Figure 2). It includes mass balance (net change in glacier total mass) measurements, glacier inventories, meteorological and hydrological observations, airborne ice thickness radar and LiDAR surveys, among others.
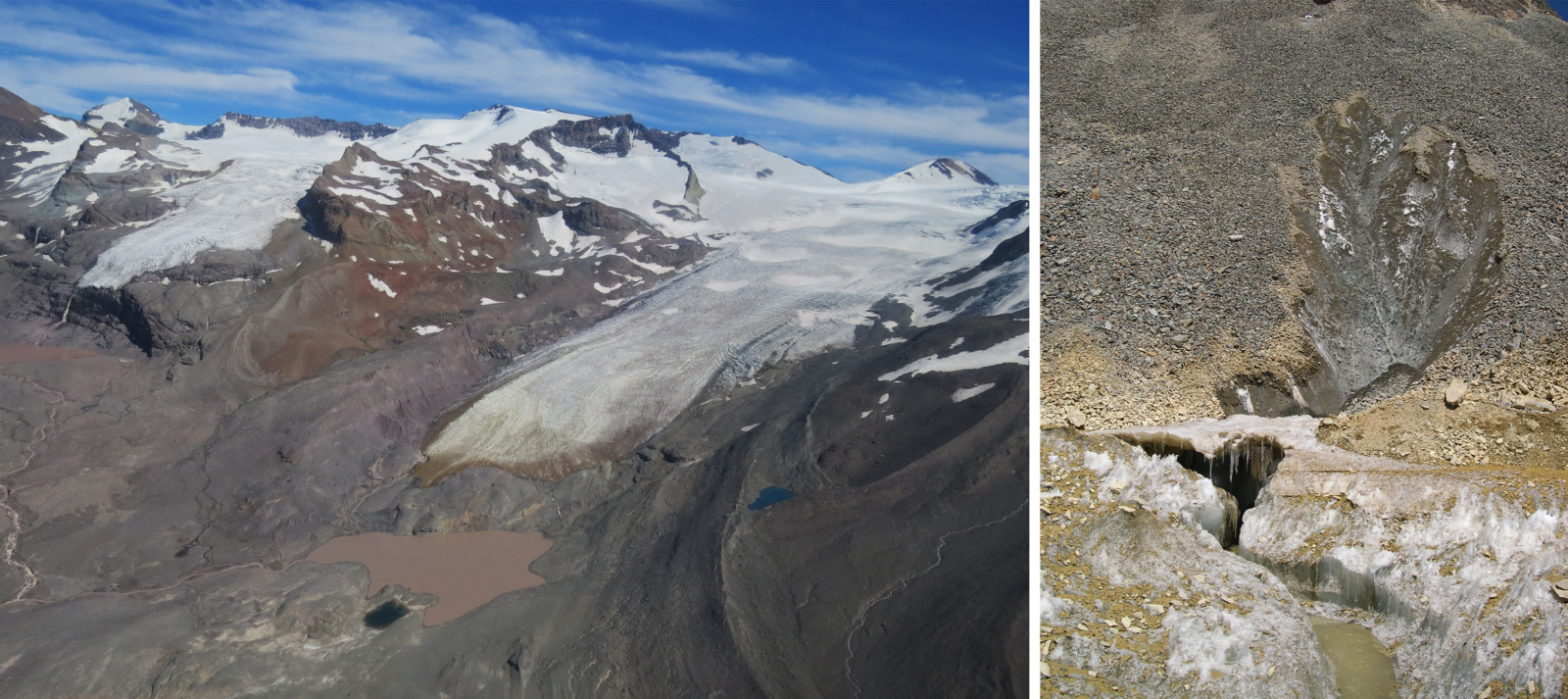
Figure 2. Left panel: Aerial view of the Olivares River sub-basin in 2017 (Olivares Beta Glacier on the left, Olivares Gamma Glacier on the right). Right panel: debris-covered ice (February 2013). Photo credit: Sebastián Cisternas.
The monitoring program has required implementing and maintaining extensive high-altitude monitoring networks, mainly composed of automatic weather stations (AWS) and mass balance stakes (Figure 3) on the Olivares River sub-basin’s glaciers. The maintenance of the installed monitoring networks has involved carrying out intense fieldwork throughout the year.
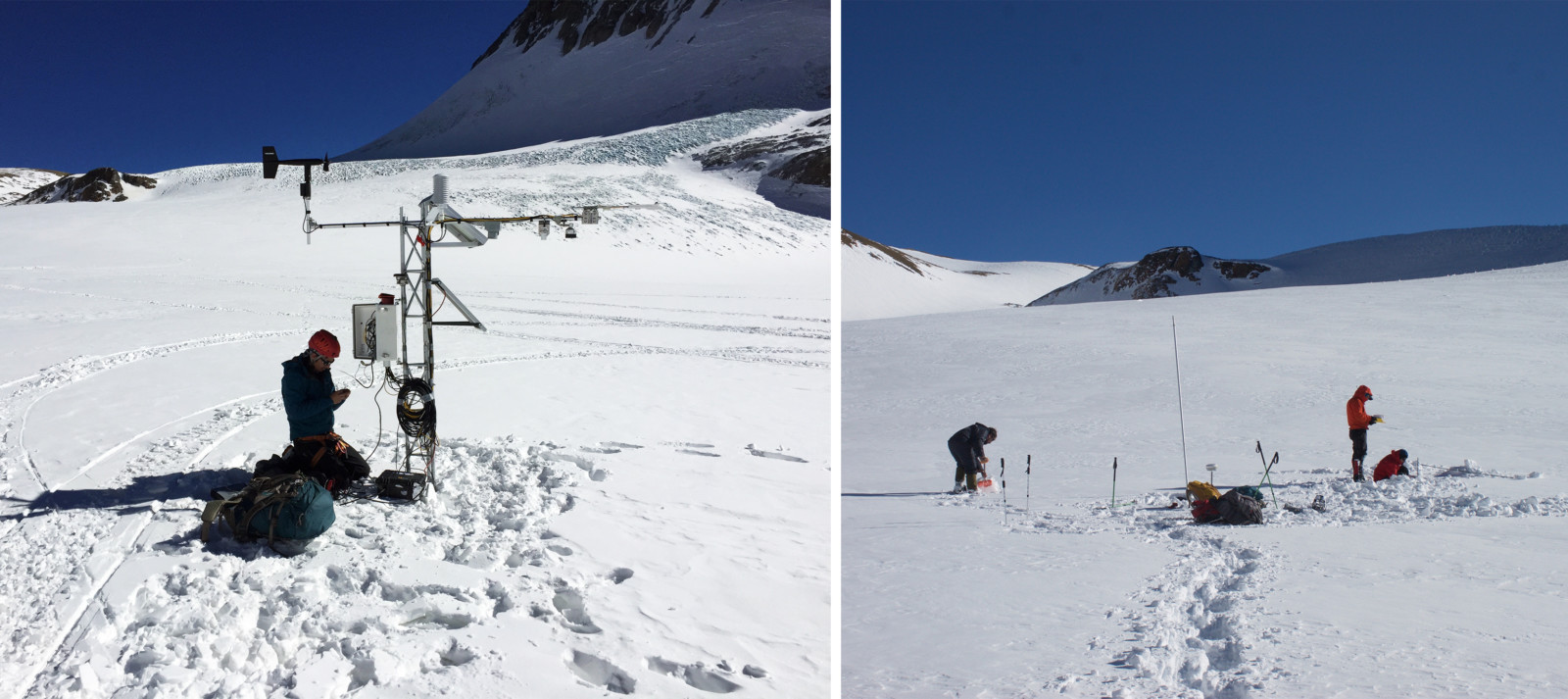
Figure 3. Left panel: AWS maintenance on the Olivares Alfa glacier (July 2017). Right panel: mass balance measurements on the Olivares Alfa glacier (July 2015), a stake is visible in the middle. Photo credit: Sebastián Cisternas.
Checking the facts
Over the years, we have witnessed first-hand the rapid retreat of the Olivares River sub-basin’s glaciers, which is not only measurable, but also obvious to the eye (Figure 4). Since 1955, the Olivares River sub-basin has lost ~35% of its glacier cover (Figure 5), with the consequent fragmentation of the main glaciers into smaller ones. Year after year, since 2013, we have measured negative mass balances, with average surface ice losses of -1.6 m reaching even more than -4 m in a very dry year.
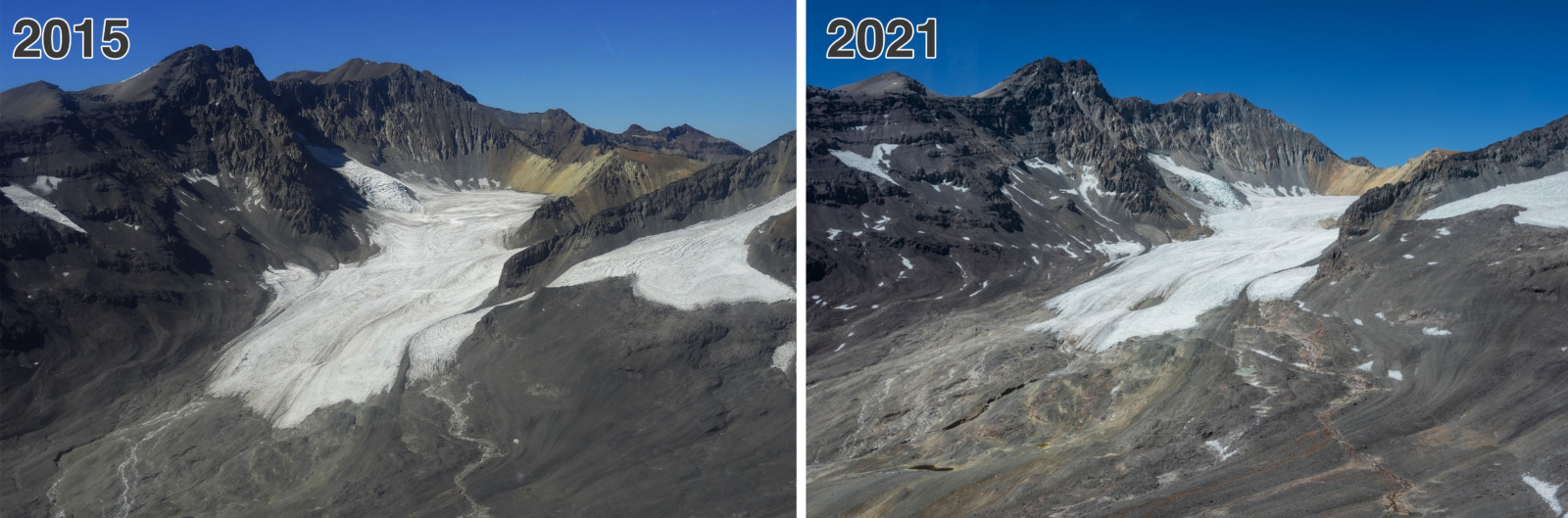
Figure 4. Comparative view of the Olivares Alfa glacier between 2015 and 2021. Photo credit: Sebastián Cisternas & Alejandro Silva
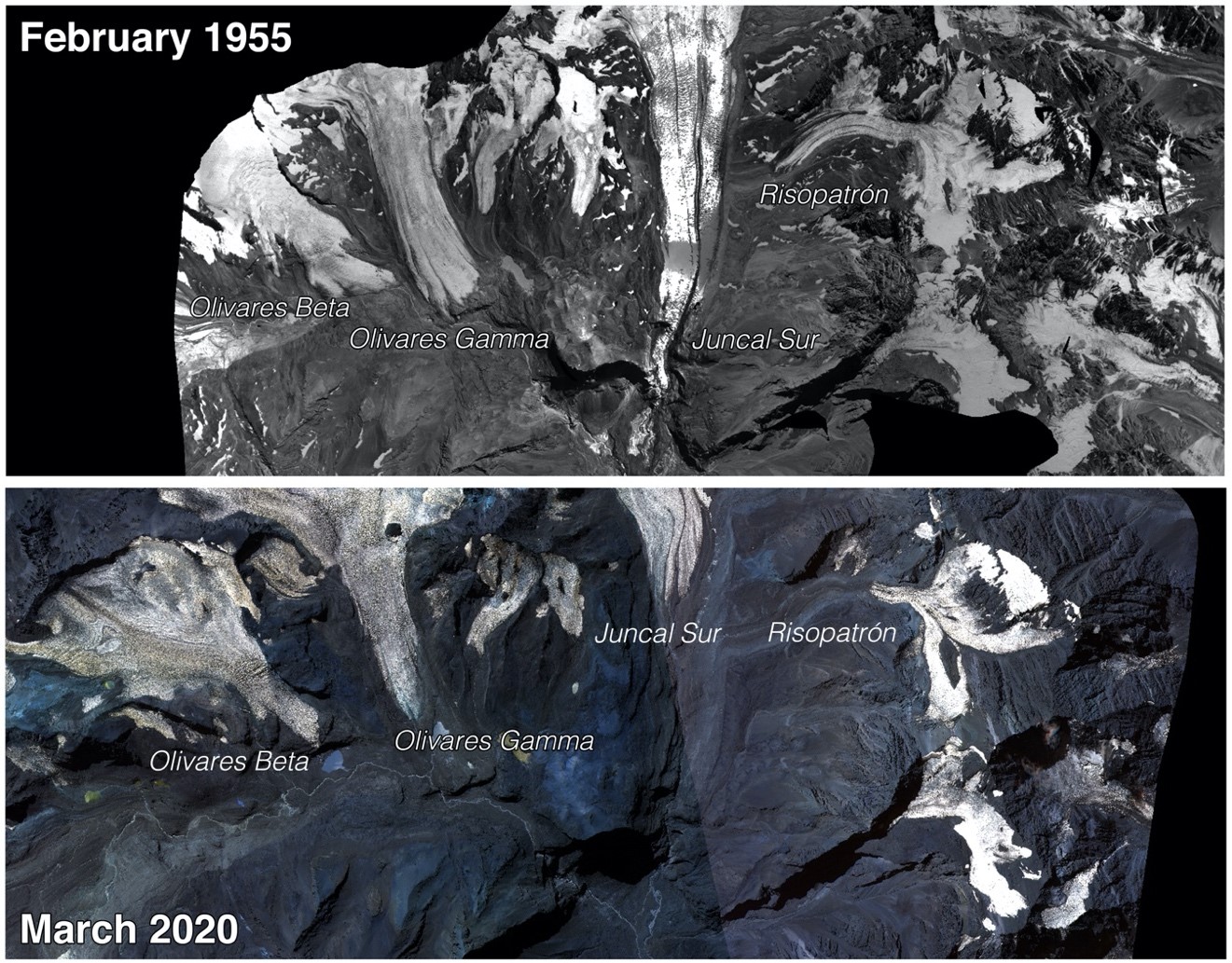
Figure 5. Changes in the extent of the Olivares Basin’s glaciers. Comparative between 1955 (Hycon photogrammetric Flight) and 2020. Figure credit: CECs.
The lack of snowfall, the high altitude that could reach the zero-degree isotherm (up to 5000 m above sea level, Figure 6), and the migration of the snow line altitude over the years (the contour line that divides snow-covered and snow-free surfaces) have given way to extended dry seasons where the ice-covered surfaces remain without the protection of the snow. Only 12% of the ice-covered area remains covered with snow in the driest periods (Figure 7). Therefore, high ice melting rates are observed all over the glaciers’ surface.
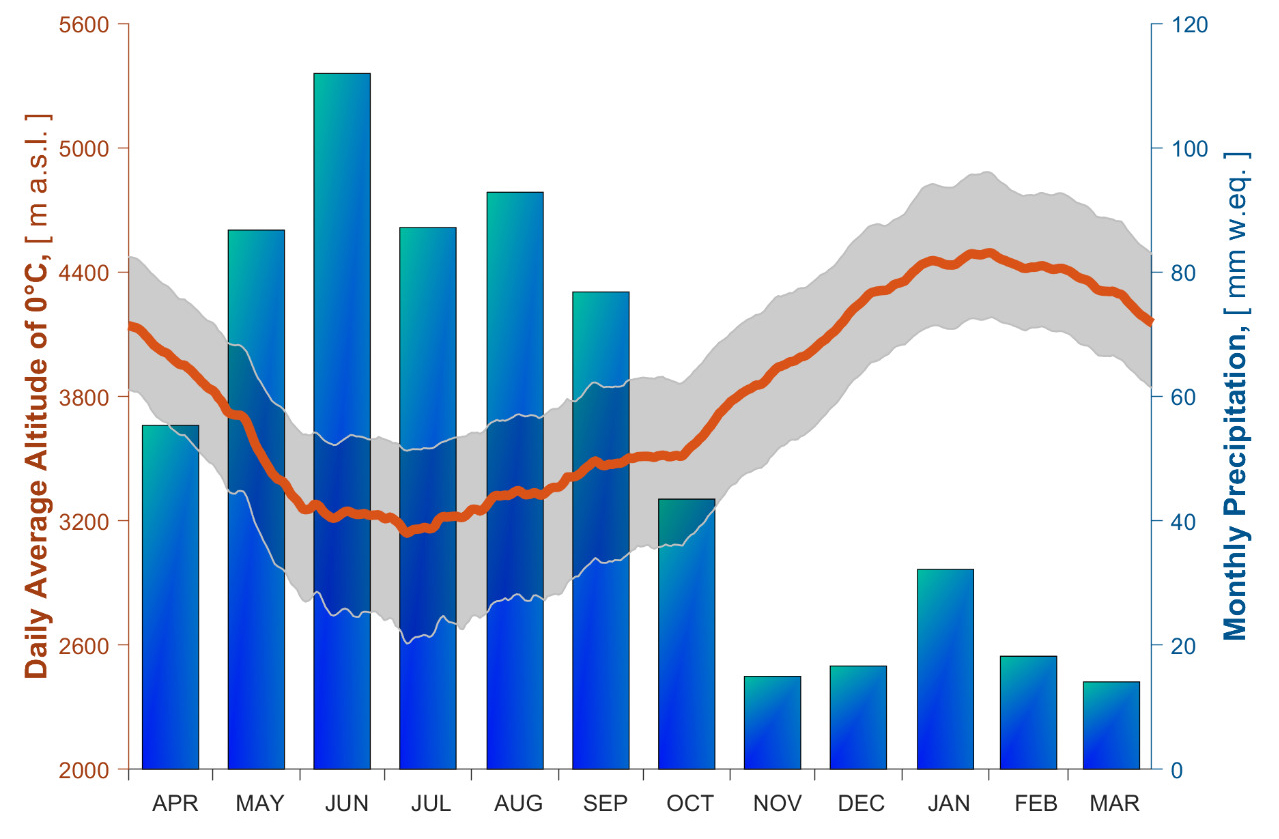
Figure 6. Measured climatic conditions between 2013 and 2021 in the Olivares River sub-basin. Figure credit: CECs.
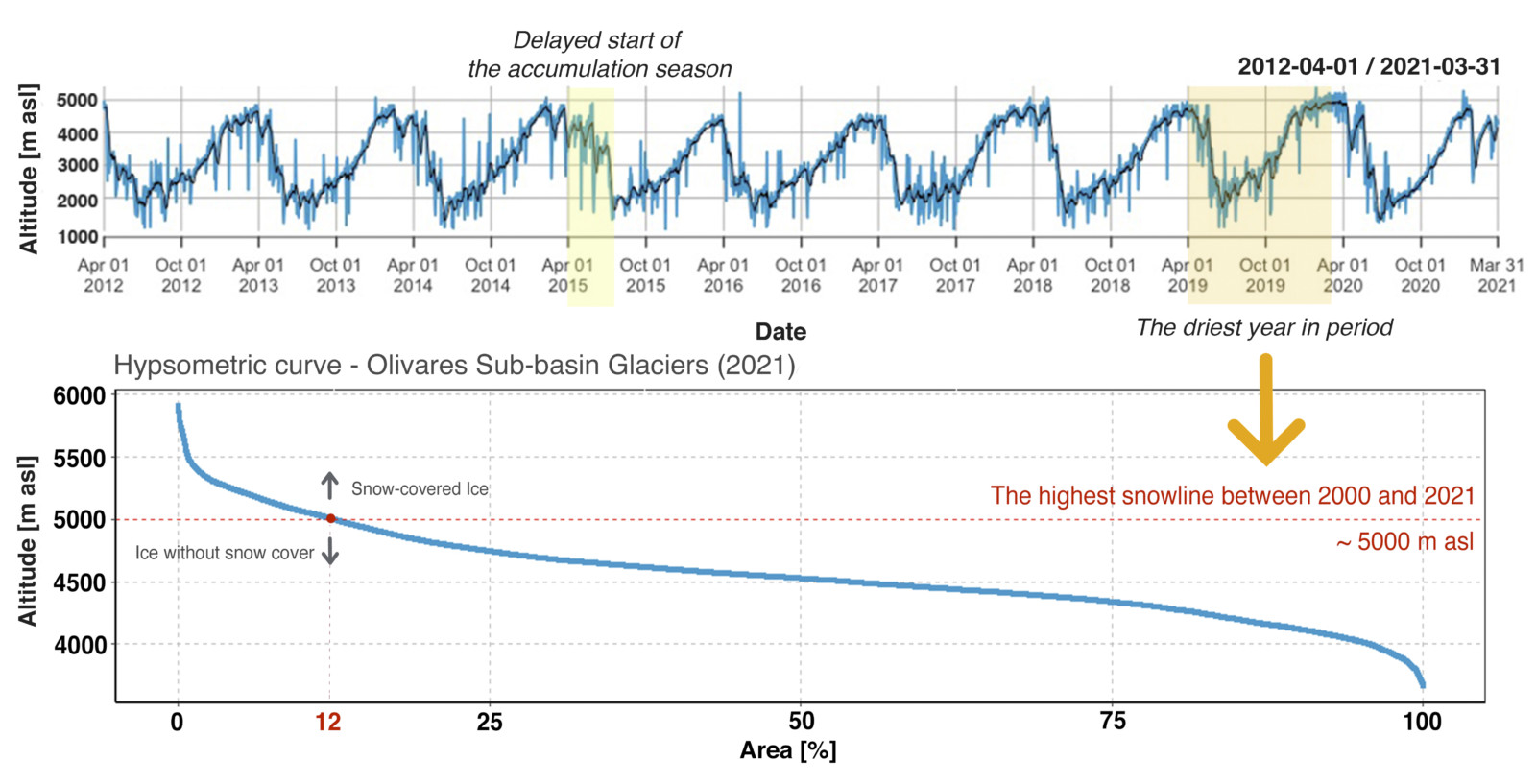
Figure 7. MODIS derived Snow Line Altitude (SLA), Maipo River Basin. Figure credit: CECs.
The monitoring challenges
Glacier imbalance and climate change imply dealing with a changing environment and therefore adapting our monitoring techniques. Each season represents different challenges, from the harsh and cold winter to the melting season, where the water on the surface makes it very difficult for us to get around.
Accumulation season
During the accumulation season (April – September) the temperature can become quite chilly, falling below -30 ºC, complicating the fieldwork, but the beautiful snowy landscape is worth it (Figure 8).
However, the sudden accumulation of snow because of seasonal atmospheric rivers in the region (Saavedra et al., 2020; Viale et al., 2018) is one of the main winter challenges (Figure 9). All the installations on the glaciers are then at risk of being buried under the snow, which would negatively affect the precision of the measurements. In consequence, we need to constantly add extra stakes and lift up the AWS after these extreme accumulation events, typically involving a lot of snow shovelling.
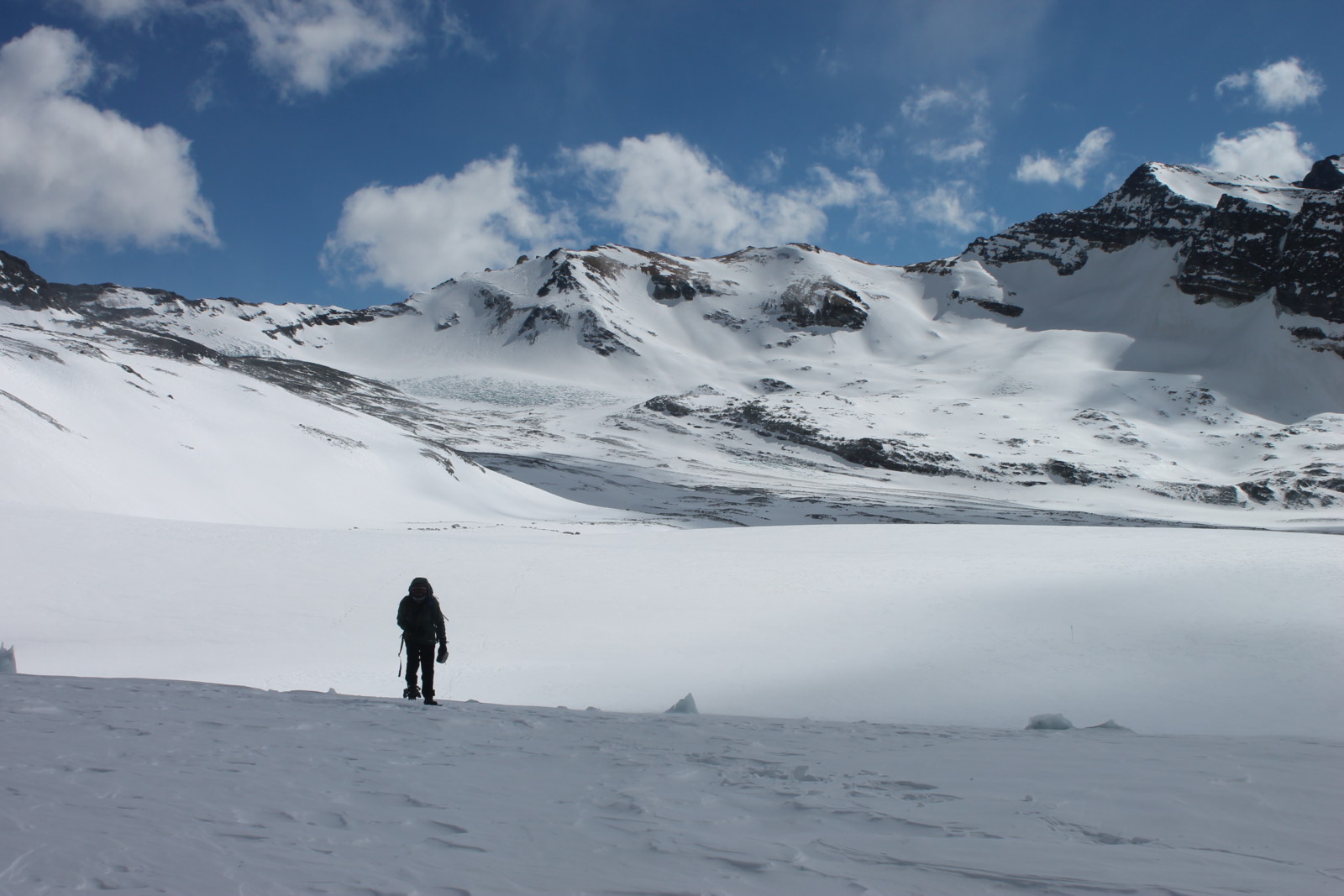
Figure 8. Snowy landscape, walking on the Olivares Alfa Glacier, July 2015. Photo credit: Sebastián Cisternas.
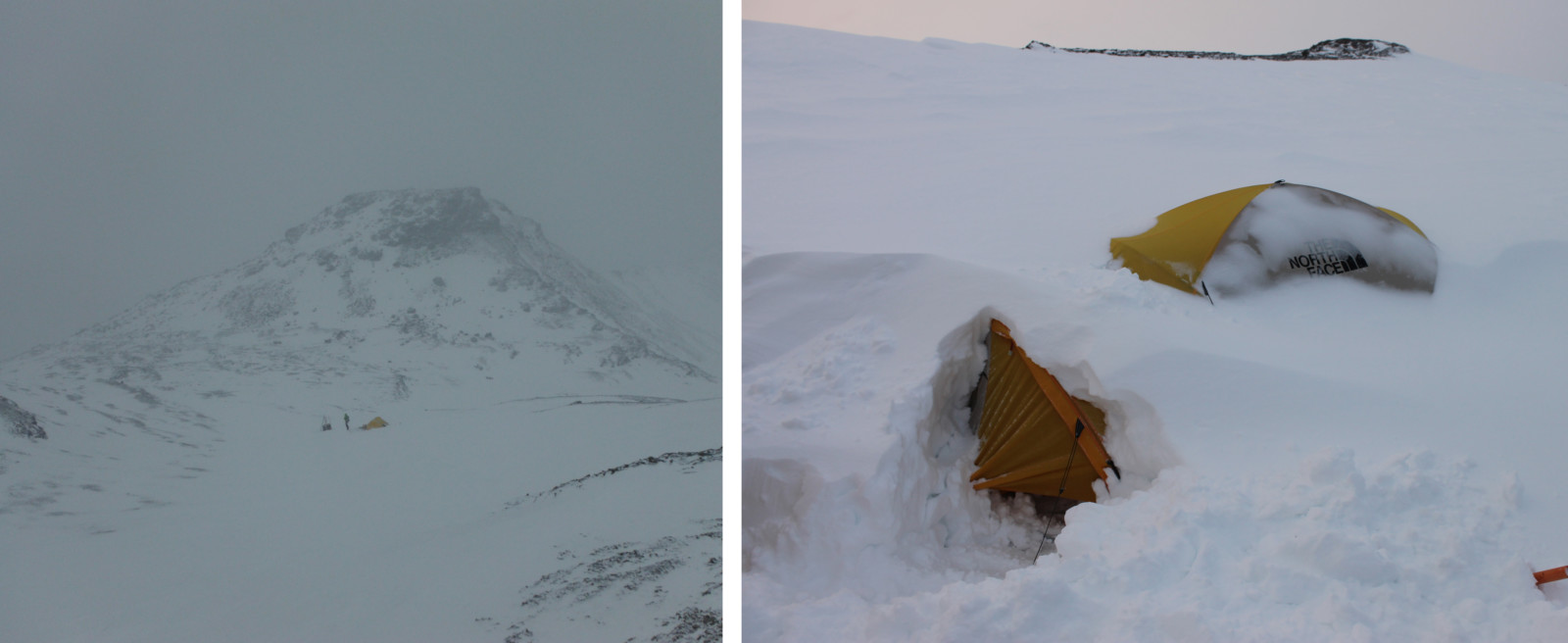
Figure 9. Our camp during a winter storm, July 2013. Photo credit: Sebastián Cisternas.
Ablation season
The ablation season (period when glaciers lose more mass than they gain, October – March) is the most challenging period of the hydrological year. The high melt rates completely transform the surface of the glaciers, removing the soft and smooth snow blanket and increasing the surface roughness. The penitentes grow (Figure 10), and there is meltwater everywhere.
The penitentes turn the glacier into a veritable maze. We have seen them reach up to 5 meters in height. The walk is slow and frustrating at times, and being able to find each instrument on the surface is a bit of a test of your character (Figure 10).
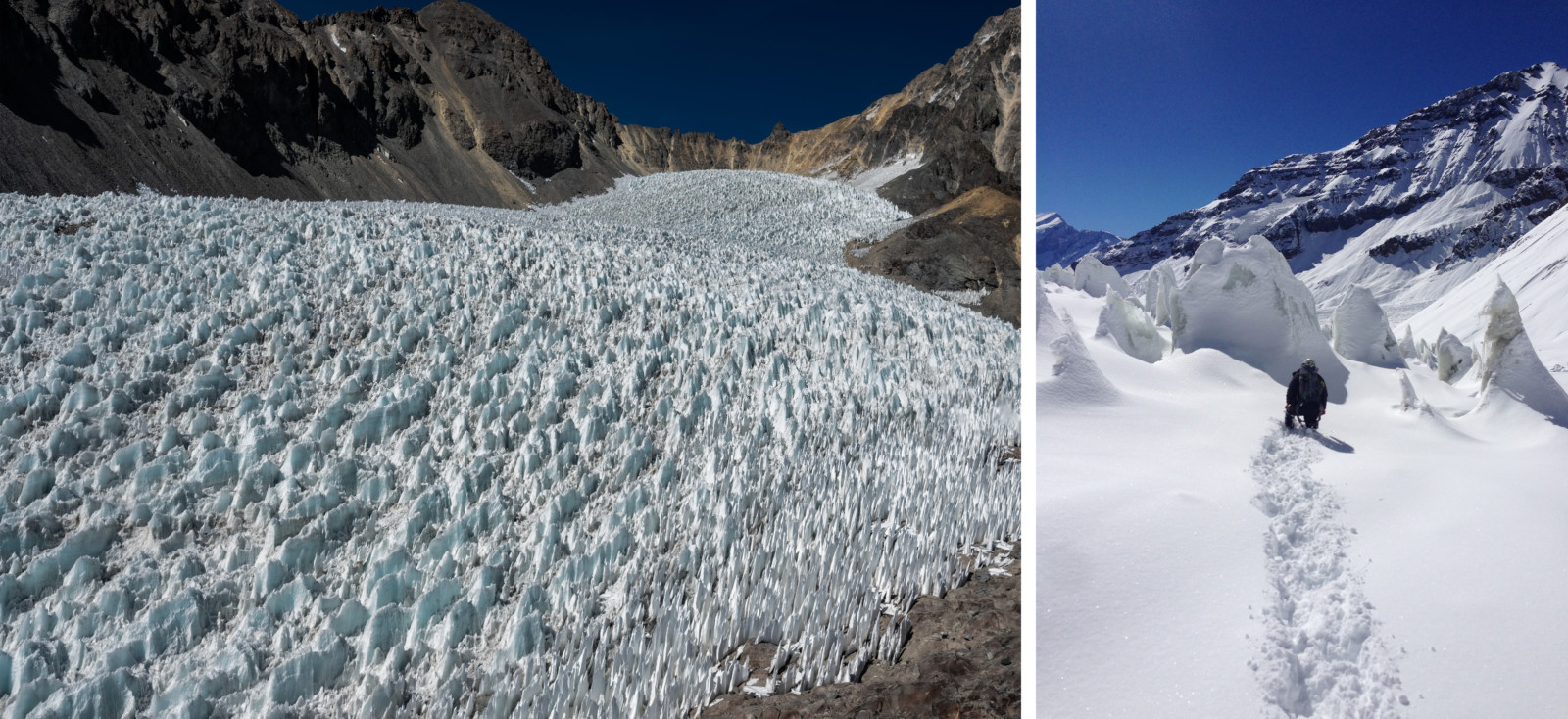
Figure 10. Left: A penitentes field on Paloma Norte glacier, January 2021. Right: Walking towards a penitentes field, Olivares Alfa, March 2015. Photo credit: Camilo Muñoz & Sebastián Cisternas.
The amount of surface water favours the formation of deep meltwater channels and ponds at the surface of the glaciers. Hence, the glaciers can look like large pools in which the penitentes swim in the summer, which makes our fieldwork even more tricky (Figure 11).
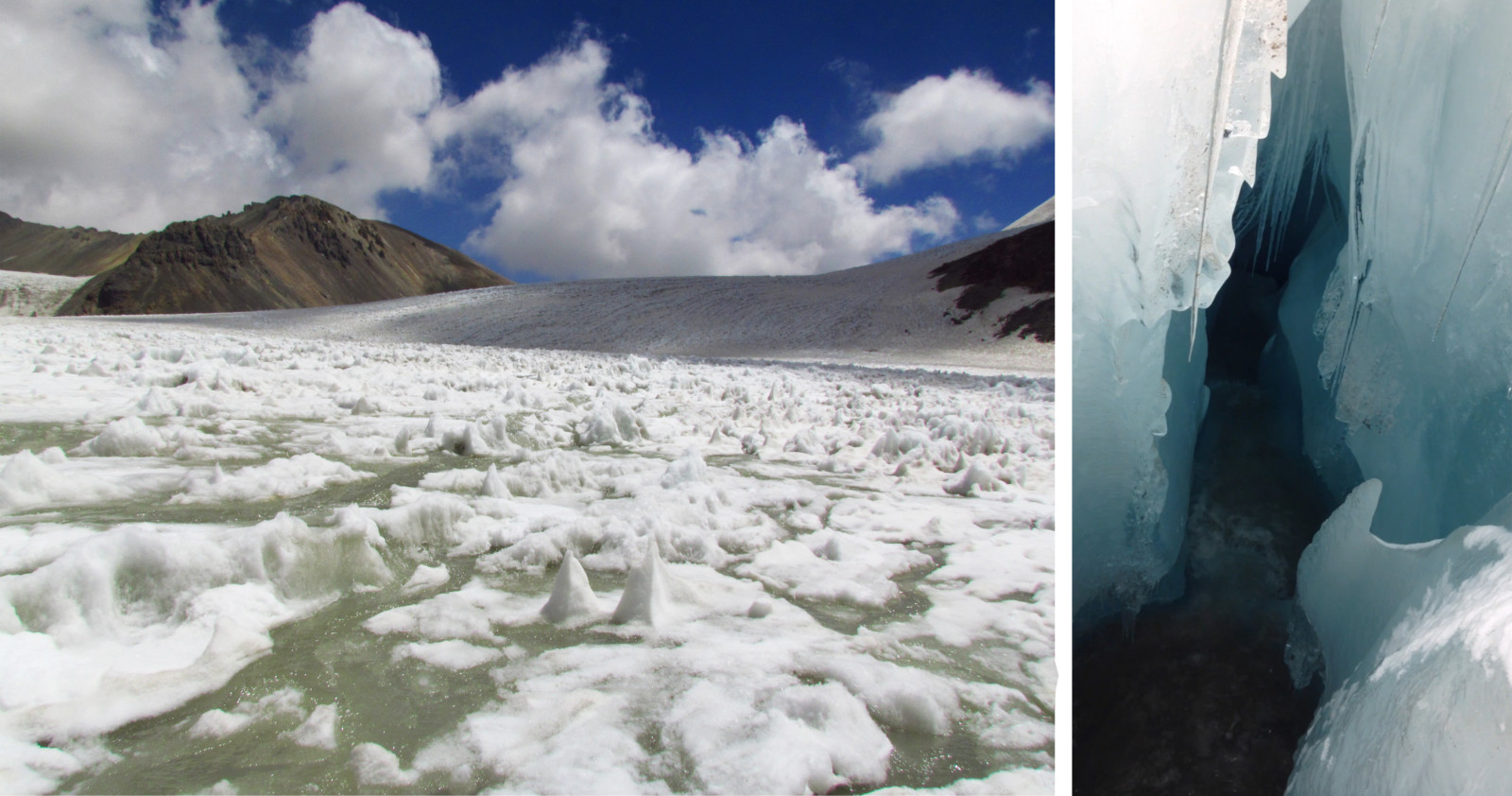
Figure 11. Left: Water on the Olivares Alfa glacier surface, January 2013. Right: A meltwater channel, March 2014. Photo credit: Sebastián Cisternas.
The ablation season has impacted our data collection in several ways, from mass balance measurements to airborne surveys. The mass balance stakes are commonly found lying down on the surface or floating in their position; the automatic weather stations cannot stay in place for long after their installation (Figure 12); and surface water diminishes the LiDAR signals, which forces us to fly closer to the surface.
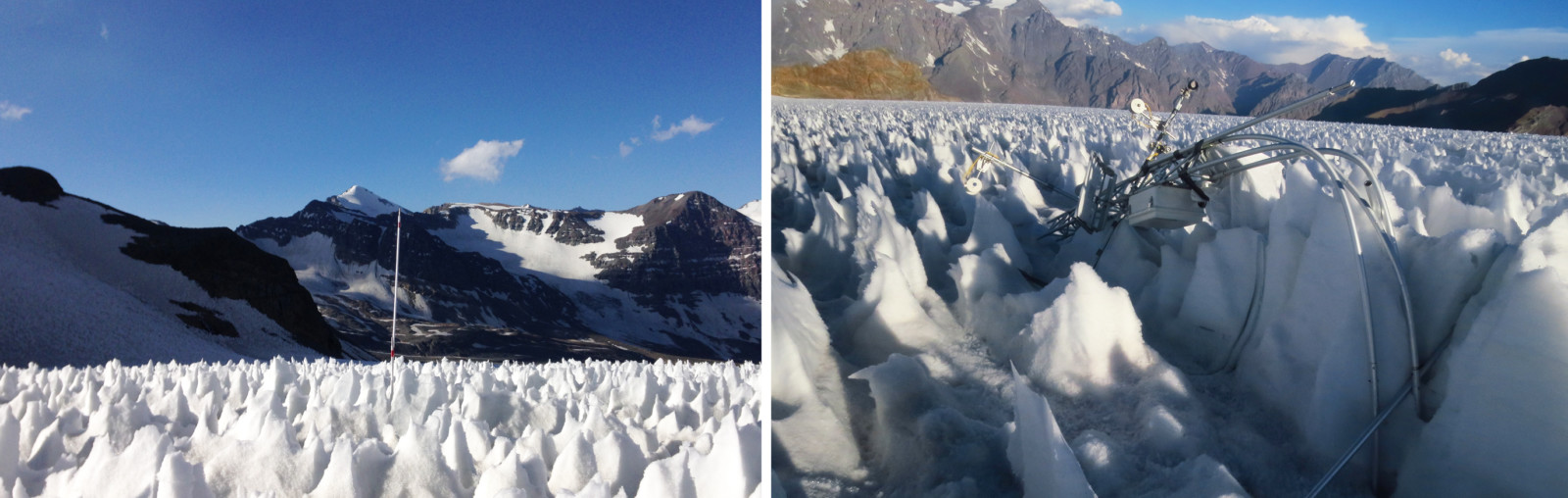
Figure 12. Left: Stake surrounded by penitentes, January 2014. Right: AWS lying down after a few weeks of its installation, January 2017. Photo credit: Sebastián Cisternas.
The climatic context and the glaciers’ state have forced and will push us to continue to look for better ways to monitor the evolution of the glaciers, to continue learning about the Andean cryosphere.
Further Reading:
- Ayala, Á., Farías-barahona, D., Huss, M., Pellicciotti, F., Mcphee, J., & Farinotti, D. (2020). Glacier runoff variations since 1955 in the Maipo River Basin, semiarid Andes of central Chile, The Cryosphere, 14, 2005–2027.
- Burger, F., Brock, B., & Montecinos, A. (2018). Seasonal and elevational contrasts in temperature trends in Central Chile between 1979 and 2015. Global and Planetary Change, 162, 136–147.
- Falvey, M., & Garreaud, R. (2009). Regional cooling in a warming world: Recent temperature trends in the southeast Pacific and along the west coast of subtropical South America (1979–2006). Journal of Geophysical Research, 114(D4), 1–16.
- Farías-Barahona, D., Ayala, Á., Bravo, C., Vivero, S., Seehaus, T., Vijay, S., et al. (2020). 60 years of glacier elevation and mass changes in the Maipo River Basin, central Andes of Chile. Remote Sensing, 12(10), 1–19.
- Garreaud, R. D., Boisier, J. P., Rondanelli, R., Montecinos, A., & Veloso-aguila, H. H. S. D. (2019). The Central Chile Mega Drought (2010 – 2018): A climate dynamics perspective, (June), 1–19.
- Malmros, J. K., Mernild, S. H., Wilson, R., Yde, J. C., & Fensholt, R. (2016). Glacier area changes in the central Chilean and Argentinean Andes 1955-2013/14. Journal of Glaciology, 62(232), 391–401.
- Saavedra, F., Cortés, G., Viale, M., Margulis, S., & McPhee, J. (2020). Atmospheric Rivers Contribution to the Snow Accumulation Over the Southern Andes (26.5° S–37.5° S). Frontiers in Earth Science, 8(July), 1–11.
- Turrel, M. (2019). El hombre que descifró los glaciares Louis Lliboutry. Aguas Andinas
- Viale, M., Valenzuela, R., Garreaud, R. D., & Ralph, F. M. (2018). Impacts of atmospheric rivers on precipitation in Southern South America. Journal of Hydrometeorology, 19(10), 1671–1687.
- Other Cryoblog posts about changes in the Chilean Andes:
Edited by Marie Cavitte
Sebastián Cisternas is a researcher at Centro de Estudios Científicos (CECs), Vadivia, Chile. He’s focused on the study of Andean glaciers and their response to climate change and local human activities. Contact email: scisternas@cecs.cl. He tweets as @scisternasg.
Camilo Muñoz is a geographer at CECs, he’s worked in the Northern and Central Andes of Chile, focusing his work on glaciological mass balance in high altitude glaciers. He is also interested in glaciovolcanic interactions in the Southern Andes. Contact email: cmunoz@cecs.cl
Claudio Bravo holds a PhD in Physical Geography from the University of Leeds, United Kingdom. Is a researcher at CECs. He carries out investigations on the response of the Andean Cryosphere to meteorological conditions and climate change. He tweets as @C_BravoLechuga. Contact email: cbravo@cecs.cl
Pablo Paredes is an atmospheric researcher at CECs. His interests are the atmospheric dynamics in complex terrain, mountain meteorology, and the interactions between the atmosphere and snow/ice in a climate change context. Contact email: pparedes@cecs.cl