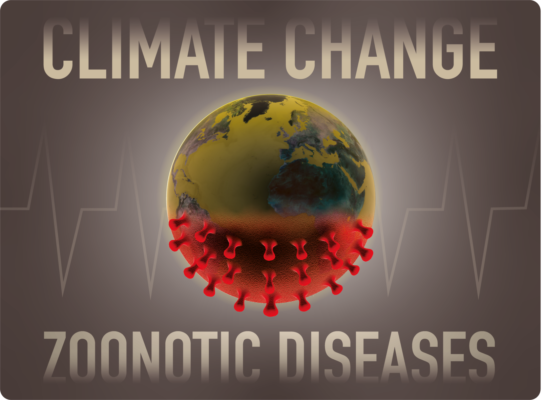
The recent coronavirus outbreak (i.e., nCovID-19; Fig. 1) has caused global panic, along with widespread travel bans, home quarantines and country-wide lockdowns. The World Health Organization (WHO) declared nCoVID-19 as a pandemic as of March 11th, 2020 (WHO, 2020). To tackle this global health crisis, scientists are attempting to synthesize a vaccine, while countries are trying to mitigate the number of infections by isolating infected patients and vulnerable populations. However, nCovID-19 is not the only zoonotic disease that has caused a major epidemic and pandemic over the past few decades. Famous examples include the Spanish flu, Zika virus, Severe Acute Respiratory Syndrome (SARS), human immunodeficiency viruses (HIV), Ebola, and Lyme disease. A natural question to ask is: Are the risks of zoonotic diseases rising in the Anthropocene due to human-induced climate change?
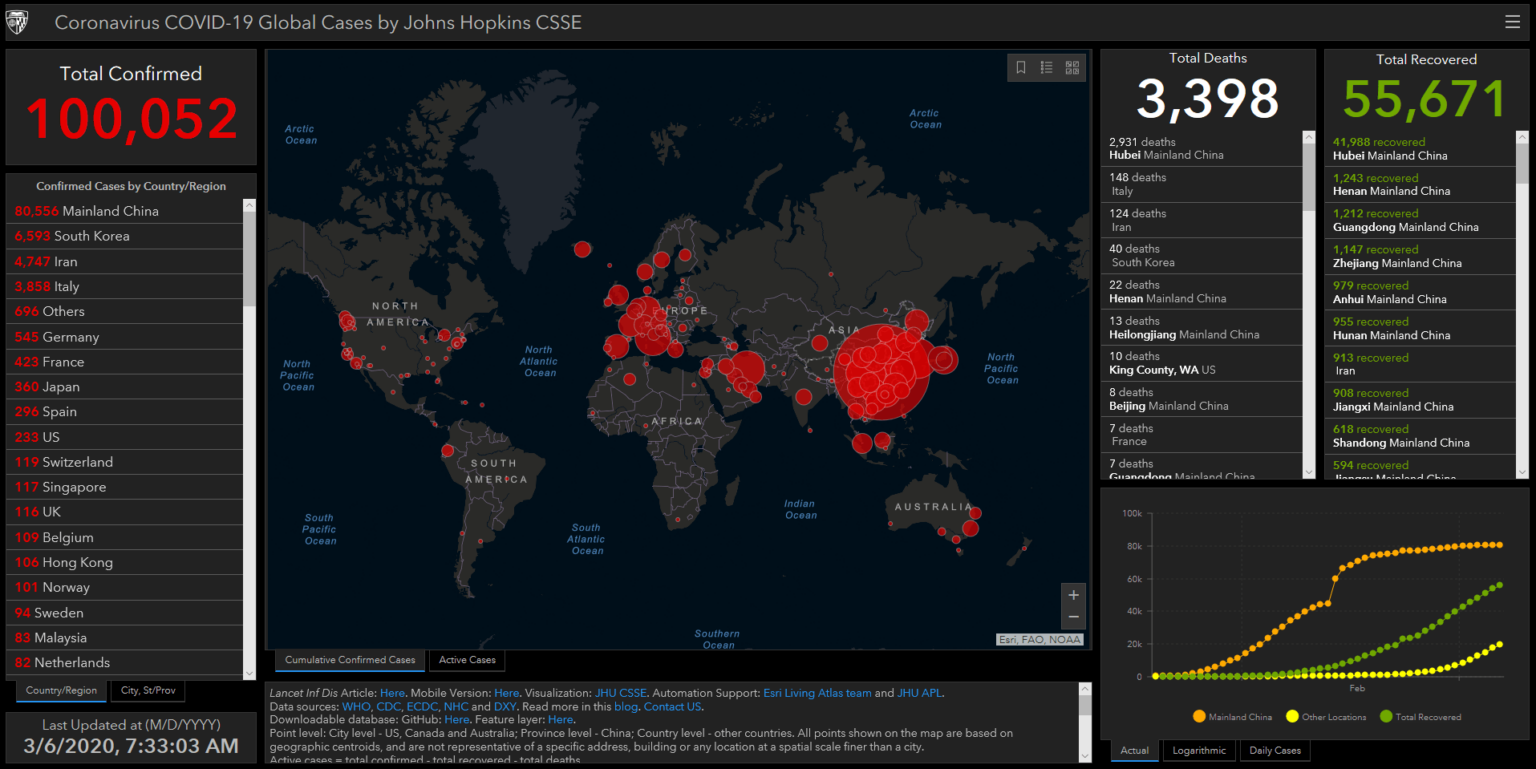
Figure 1. 2019 coronavirus tracker released by the Center for Systems Science and Engineering (CSSE) at Johns Hopkins University (2020). Click the figure to access it with up-to-date numbers.
What is a zoonotic disease?
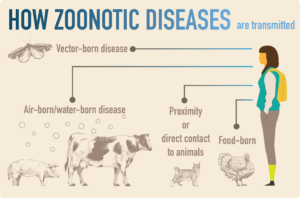
Figure 2. Zoonotic diseases and their mechanisms of transmission (figure made by Ichiko Sugiyama; information from the London School of Hygiene and Tropical Medicine (2017); Thornton, 2017).
Zoonotic diseases are a class of common infectious diseases that spread from animals to humans, either by bacteria, viruses, parasites or fungi. As seen in Figure 2, there are numerous ways in which animal-circulated pathogens can be passed to humans. Airborne diseases, like influenza, are pathogens that can be transmitted by close proximity to animals. Vector-born diseases, such as malaria or Lyme disease, can be transmitted by insects, e.g. mosquitos or ticks. Food-borne diseases, such as salmonella, can be transmitted via consumption of animal products, e.g. chicken or fish. A final transmission pathway is through direct contact with animals, such as animal bites or touching of infected animals (including wildlife) e.g. to rabies, HIV, Ebola, SARS, nCovID-19 (?). Thus, paths of zoonotic diseases are multi-faceted. A list of zoonotic diseases can be found in Table 1.
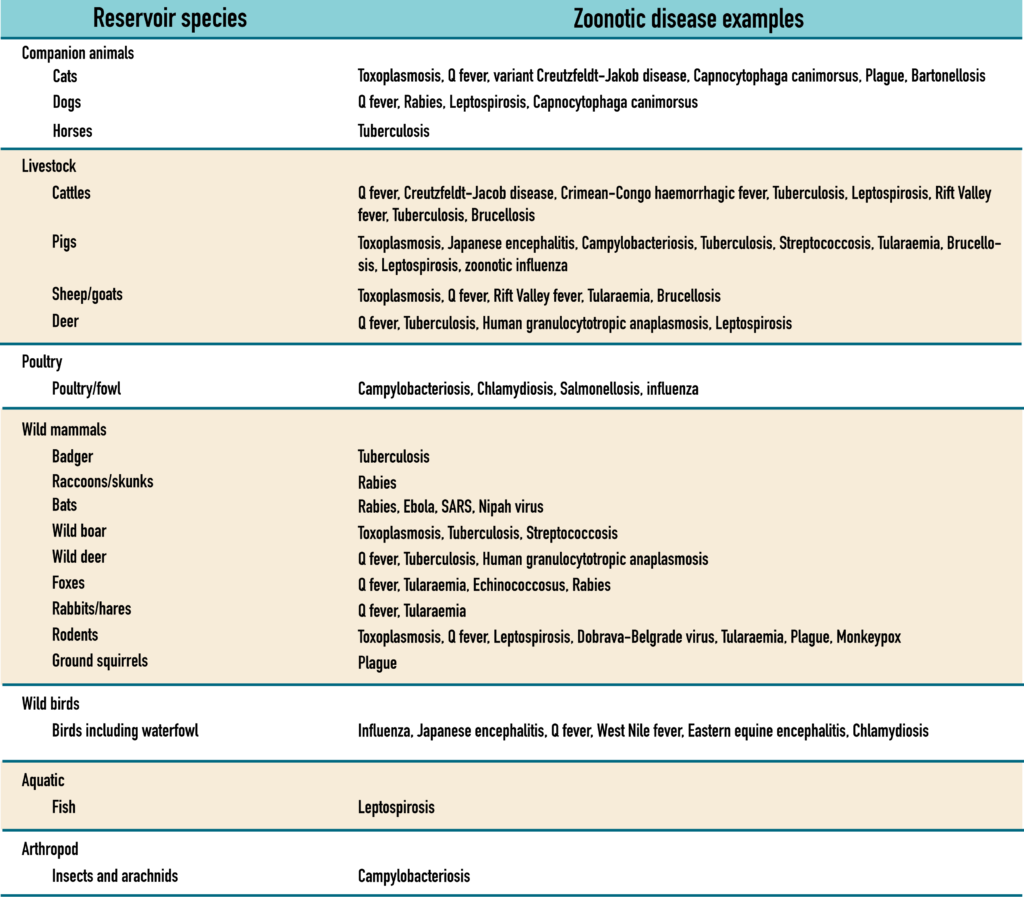
Table 1. Potential disease reservoir reported by Goodwin et al., (2012) for Zoonotic diseases (the table excludes vector species).
It is becoming clear that humans are inducing significant changes to the global environment and the ecosystems within it (Watson et al., 2002; Boillat and Ifejika Speranza, 2019). The emergence of zoonotic diseases in the past century is partly due to the rise in human population along with increases in both travel and trade (Fig. 3). These changes have prompted the re-distribution and increased trafficking of animal species which has led to an increase in the frequencies of human and wild animal contact (Jones et al., 2013; Pecl et al., 2017; Fig. 3A and 3B). Increases to human population also bears additional consequences, such as urbanization, which can drastically change the landscape of human and wild animal interactions. In the following section, we will be discussing how the effects of anthropogenic climate change can manifest in the emergence of zoonotic diseases.
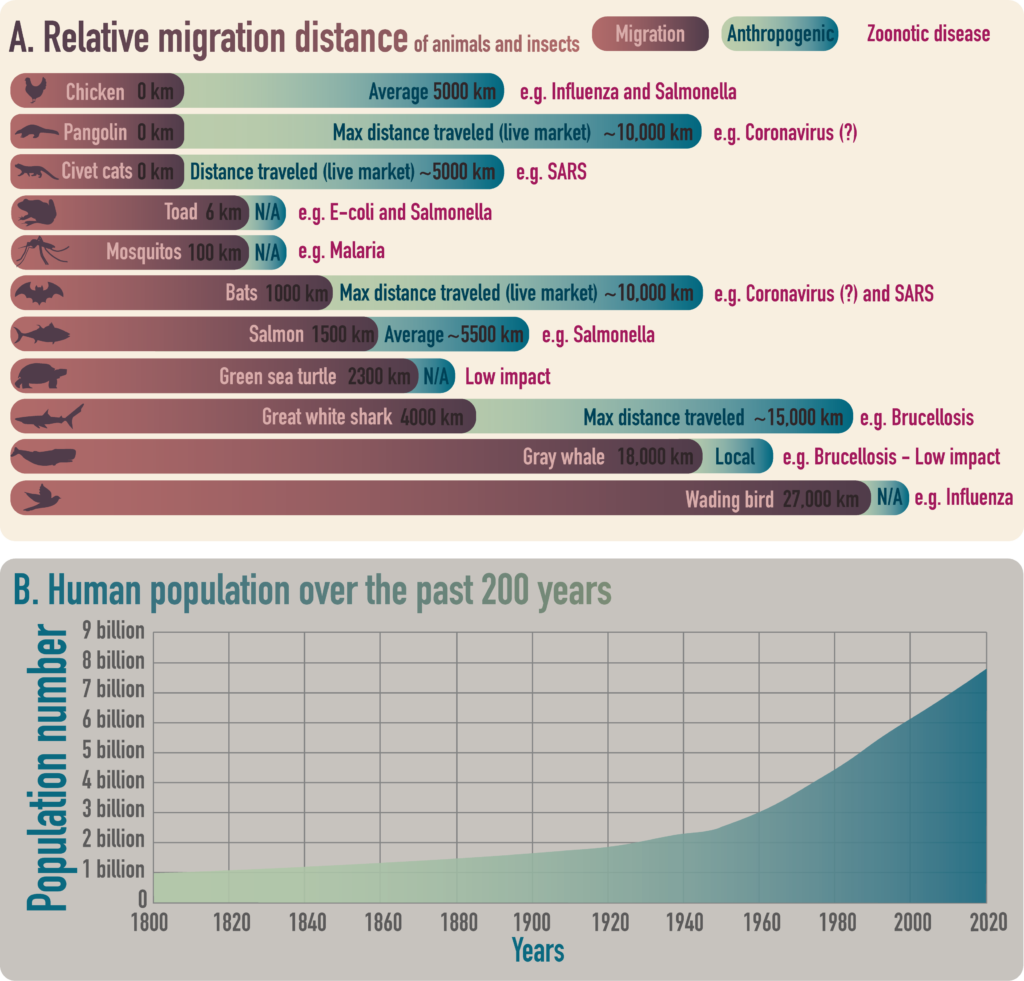
Figure 3. A. Infographics of various animals and insects and their natural migration distance (color maroon; after Altizer et al., 2011), re-distribution of species via trading (color: blue; data retrieved from food mile calculator (2020), Wiles et al. (1989), African Wildlife Foundation (2020), and Animalia (2020)), and potential carrier of disease (color: pink; Goodwin et al., 2012). B. Human population has drastically increased over the past ~200 years (data retrieved from the Our World in Data, 2020).
Climate change and the emergence of zoonotic diseases
The most recent Intergovernmental Panel on Climate Change (IPCC) report outlines that Earth could see global mean surface temperatures rise by up to 4 ˚C by the end of this century (RCP 8.5 scenario). Such warming may not seem so significant, but it is becoming clear that even 1 °C above pre-industrial levels implies large environmental changes: from melting ice-sheets to changes in the patterns of precipitation and drought (e.g. Wilhite, 2000; Pollard et al., 2015; IPCC AR5, 2018; Myhre et al., 2019). Gradually, scientists have been working to understand the effects of climate change on elements beyond the conventionally-defined climate system, such as vegetation, marine ecosystems or land surface (Fig. 4).
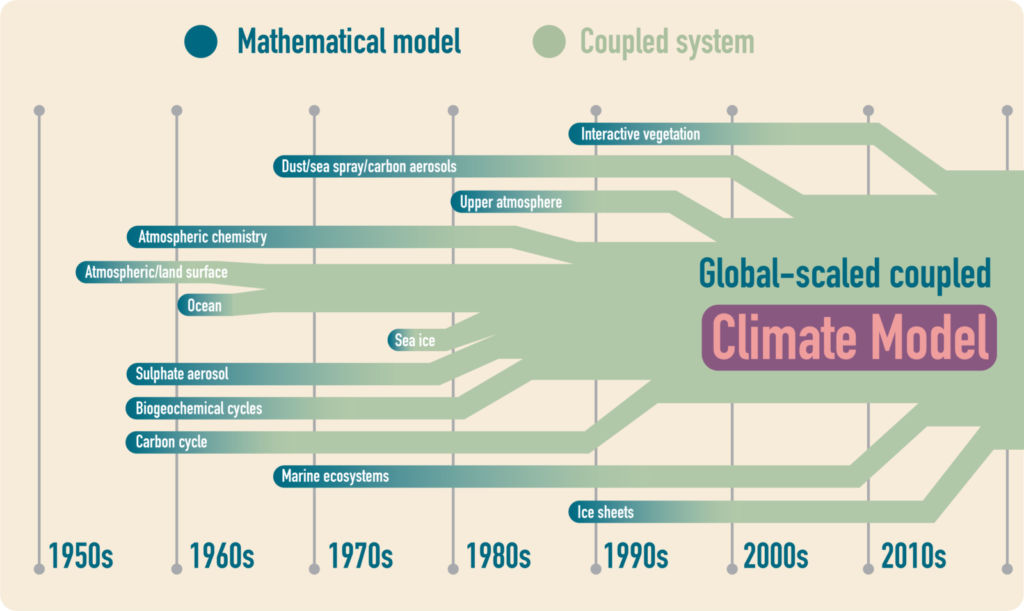
Figure 4. At different stages of the IPCC reports (total of six assessment reports), many scientists started incorporating different relevant parameters into the global-scaled coupled climate models, sometimes termed Earth System Models (Carbon Brief; original graphic by Rosamund Pearce; based on the work of Dr Gavin Schmidt; figure made by Ichiko Sugiyama).
One of the potential effects of climate, which is not often advertised, is its role in the emergence or re-emergence of zoonotic diseases (Fig. 5) and over the past decade, epidemiologists and climate scientists have been exploring such links (Morse, 2001; McMichael, 2004; Harper and Armelagos, 2010; Goodwin et al., 2012; Jones et al., 2013). There has been a rise in the distances species typically migrate due to climate change (animals and human), and it is argued that this trend will continue over time (Bates, 2002; Besthorn and Meyers, 2010; Pecl et al., 2017; IPCC AR5 WGII, 2014). Changes in migratory patterns can result in modified interactions between wildlife and humans (Despommier et al., 2006; Jones et al., 2013). Furthermore, these trends are predicted to be compounded when coupled to extreme weather events that are a consequence of anthropogenic climate change (IPCC AR5 WGII Ch. 11, 2014).
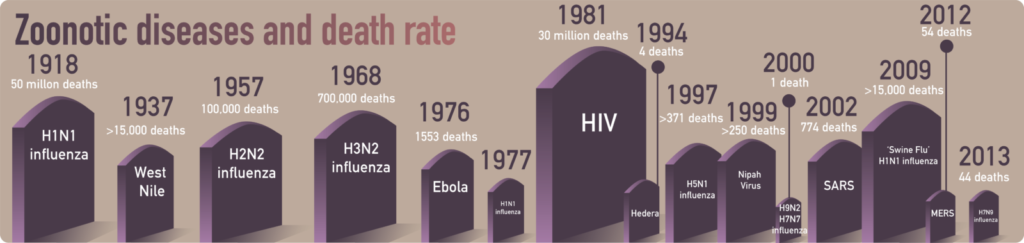
Figure 5. Data taken from Bean et al., (2013) on emergence of zoonosis (figure made by Ichiko Sugiyama).
Despite uncertainties in predicting the future effects of anthropogenic climate change on human and animal health, many epidemiologists agree that the changes in climate and ecotones, a transitional area between two organisms, has a crucial role to play in regulating the emergence and re-emergence of zoonotic diseases. This argument is well supported from looking back over the past few decades where: the influence of climate through temperature changes has been shown to increase malaria transmission (Paaijmans et al., 2010), changes in annual temperature and precipitation patterns have been shown to have links to the preponderance of dengue fever through the rise in Asian tiger mosquito populations (Ogden et al., 2014), reports have linked climate change to a rise in tick-born disease, such as Lyme disease (e.g. Okuthe and Buyu, 2006; Lukan et al., 2010; Tokarevich et al., 2011; Andreassen et al., 2012; Estrada-Peña et al., 2012; Jaenson et al., 2012), and finally, the Hanta virus has been tied to seasonal and inter-annual variability in climate, as well as a rise in temperature, precipitation and humidity (Klempa, 2009; Fang et al., 2010; Liu et al., 2011).
Earth has seen dramatic changes in climate over 4 billion years. The question remains: How will climate change affect humanity in the near future?
The United States department of defense has classified climate change as a threat multiplier (U.S. department of defense, 2014). Furthermore, the cost and death toll from natural disasters and pandemics are very high and can impact the global economy and increase socioeconomic divides (Fig. 5 and 6). The problem is not only that anthropogenic climate change will induce dramatic changes in the Earth’s biogeochemical cycles, but also that humans’ ability to adapt to the rapid changes that they have imposed will be incredibly costly.
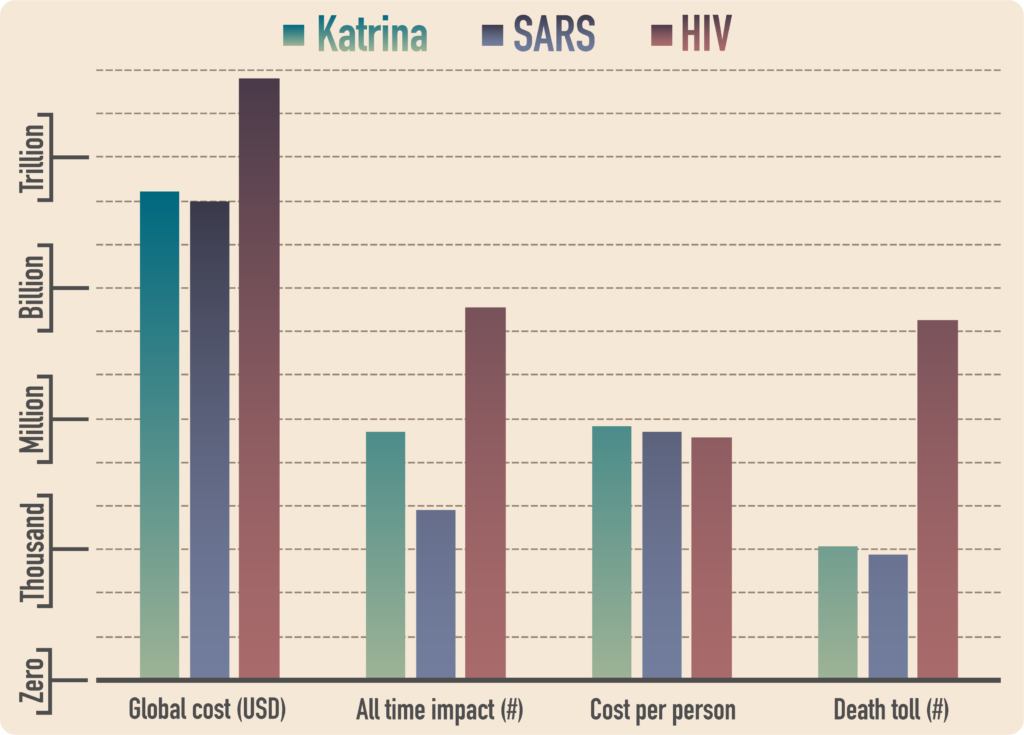
Figure 6. Comparison of approximate global cost, all time impact (number of people affected), cost per person and death toll of hurricane Katrina (data retrieved from the Data Center (2016)), SARS (data retrieved from Qiu et al., 2018) and HIV (data retrieved from Our World in Data (2019)). The approximate global cost was calculated using order of magnitude estimation (infected minus deceased multiplied by the cost per person); figure made by Ichiko Sugiyama.
Research on the relationship between climate and diseases has seen an exponential growth over the past few decades (Fig. 7), reflecting the urgency to understand the potential links between climate change and zoonotic diseases, and this trend will likely increase in the future. While climate scientists and epidemiologists face many uncertainties in understanding climate patterns and how diseases emerge and spread, links and feedbacks between these two seemingly disparate fields are emerging. It is often difficult to link a specific extreme weather event, such as a hurricane, to the effects of anthropogenic climate change. However, the use of numerical models and multi-decadal data allows us to determine with a higher degree of confidence the link between a class of extremes, such as category 4 and 5 hurricanes, and climate change. The same can be said about zoonotic diseases. We may struggle to tell whether the outbreak of nCovID-19 was accelerated by factors related to climate change or not, but by utilizing scientific tools and models along with interdisciplinary efforts, we may uncover critical links between anthropogenic climate change and zoonotic diseases. Thus, society should be mindful of the changes humans are making to the Earth system, and should prepare to address the potential effects of climate change and anthropogenic activities on the occurrence and frequency of pandemic-level events.
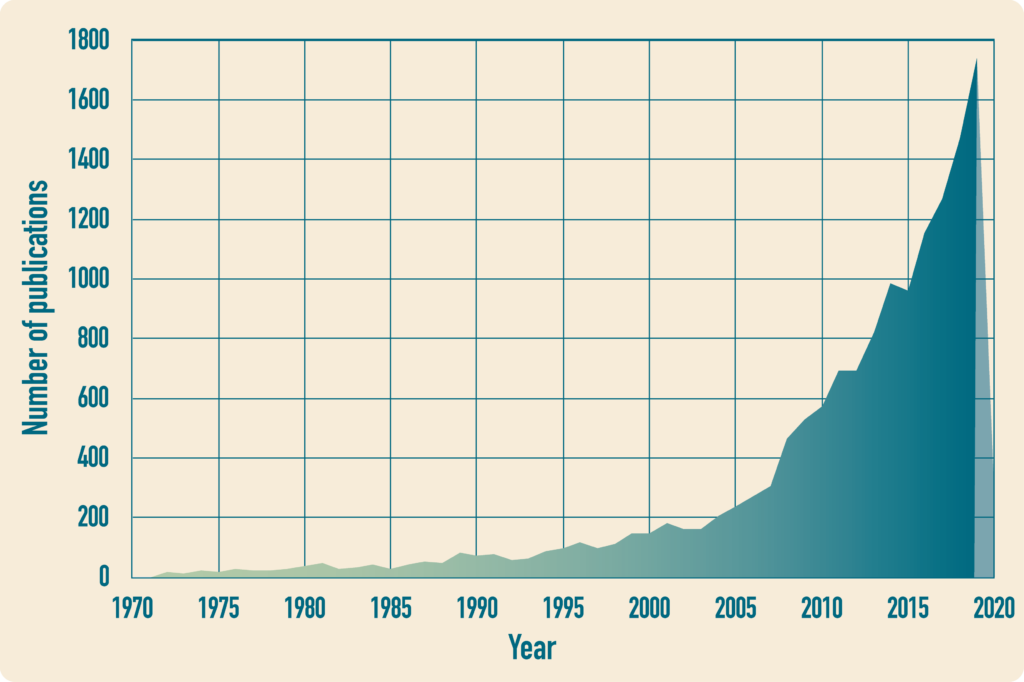
Figure 7. Number of scientific papers containing both climate and diseases in their title has been increasing exponentially in the past few decades (data retrieved from dimensions.ai, 2020).
This post has been edited by J. Bösken, G. Messori, and C. Nehme.
REFERENCES African Wildlife Foundation (2020). Retrieved from https://www.awf.org/wildlife conservation/pangolin. Accessed March 11th, 2020. Altizer, S., Bartel, R., & Han, B. A. (2011). Animal migration and infectious disease risk. science, 331(6015), 296-302. Andreassen, A., Jore, S., Cuber, P., Dudman, S., Tengs, T., Isaksen, K., ... & Vainio, K. (2012). Prevalence of tick borne encephalitis virus in tick nymphs in relation to climatic factors on the southern coast of Norway. Parasites & vectors, 5(1), 177. Animalia (2018) Asian Palm Civet. Retrieved from http://animalia.bio/asian-palm-civet. Accessed March 11th, 2020. Bates, D. C. (2002). Environmental refugees? Classifying human migrations caused by environmental change. Population and environment, 23(5), 465-477. Bean, A. G., Baker, M. L., Stewart, C. R., Cowled, C., Deffrasnes, C., Wang, L. F., & Lowenthal, J. W. (2013). Studying immunity to zoonotic diseases in the natural host—keeping it real. Nature Reviews Immunology, 13(12), 851-861. Besthorn, F. H., & Meyer, E. E. (2010). Environmentally Displaced Persons. Critical Social Work, 11(3). Boillat, S., & Ifejika Speranza, C. (2019). IPBES Global Assessment Report on Biodiversity and Ecosystem Services. Chapter 3. Assessing progress towards meeting major international objectives related to nature and nature’s contributions to people. Carbon Brief (2018, January 15) Q&A: How do climate models work? Retrieved from https://www.carbonbrief.org/qa-how-do-climate-models-work. Accessed March 11th, 2020. The Center for Systems Science and Engineering (CSSE) at Johns Hopkins University (2020) Coronavirus COVID-19 Global Cases by Johns Hopkins CSSE. Retrieved from https://gisanddata.maps.arcgis.com/apps/opsdashboard/index.html#/bda7594740fd40299423467b48e9ecf6. Accessed March 11th, 2020. The Data Center (2016, August 26) Facts for Features: Katrina Impact. Retrieved from https://www.datacenterresearch.org/data-resources/katrina/facts-for-impact/. Accessed March 11th, 2020. Despommier, D., Ellis, B. R., & Wilcox, B. A. (2006). The role of ecotones in emerging infectious diseases. EcoHealth, 3(4), 281-289. Dimensions. Keywords used: Climate and Zoonotic disease. Retrieved from https://app.dimensions.ai/analytics/publication/overview/timeline? search_text=Climate%20disease&search_type=kws&search_field=text_search&year_from=1971&year_to=2020. Accessed March 11th, 2020. Estrada-Peña, A., Ayllón, N., & De La Fuente, J. (2012). Impact of climate trends on tick-borne pathogen transmission. Frontiers in physiology, 3, 64. Fang, L. Q., Wang, X. J., Liang, S., Li, Y. L., Song, S. X., Zhang, W. Y., ... & Yang, H. (2010). Spatiotemporal trends and climatic factors of hemorrhagic fever with renal syndrome epidemic in Shandong Province, China. PLoS Negl Trop Dis, 4(8), e789. Food miles calculator (2017) Information retrieved for chicken, sharks and salmon. Retrieved from https://www.foodmiles.com/. Accessed March 11th, 2020. Goodwin, R., Schley, D., Lai, K. M., Ceddia, G. M., Barnett, J., & Cook, N. (2012). Interdisciplinary approaches to zoonotic disease. Infectious Disease Reports, 4(2). Harper, K., & Armelagos, G. (2010). The changing disease-scape in the third epidemiological transition. International journal of environmental research and public health, 7(2), 675-697. IPCC, 2014: Climate Change 2014: Impacts, Adaptation, and Vulnerability. Part B: Regional Aspects. Contribution of Working Group II to the Fifth Assessment Report of the Intergovernmental Panel on Climate Change [Barros, V.R., C.B. Field, D.J. Dokken, M.D. Mastrandrea, K.J. Mach, T.E. Bilir, M. Chatterjee, K.L. Ebi, Y.O. Estrada, R.C. Genova, B. Girma, E.S. Kissel, A.N. Levy, S. MacCracken, P.R. Mastrandrea, and L.L. White (eds.)]. Cambridge University Press, Cambridge, United Kingdom and New York, NY, USA, pp. 688. IPCC, 2018: Global Warming of 1.5°C. An IPCC Special Report on the impacts of global warming of 1.5°C above pre-industrial levels and related global greenhouse gas emission pathways, in the context of strengthening the global response to the threat of climate change, sustainable development, and efforts to eradicate poverty [V. Masson-Delmotte, P. Zhai, H. O. Pörtner, D. Roberts, J. Skea, P.R. Shukla, A. Pirani, W. Moufouma-Okia, C. Péan, R. Pidcock, S. Connors, J. B. R. Matthews, Y. Chen, X. Zhou, M. I. Gomis, E. Lonnoy, T. Maycock, M. Tignor, T. Waterfield (eds.)]. Jaenson, T. G., Jaenson, D. G., Eisen, L., Petersson, E., & Lindgren, E. (2012). Changes in the geographical distribution and abundance of the tick Ixodes ricinus during the past 30 years in Sweden. Parasites & vectors, 5(1), 8. Jones, B. A., Grace, D., Kock, R., Alonso, S., Rushton, J., Said, M. Y., ... & Pfeiffer, D. U. (2013). Zoonosis emergence linked to agricultural intensification and environmental change. Proceedings of the National Academy of Sciences, 110(21), 8399-8404. Klempa, B. (2009). Hantaviruses and climate change. Clinical Microbiology and Infection, 15(6), 518-523. Liu, X., Jiang, B., Gu, W., & Liu, Q. (2011). Temporal trend and climate factors of hemorrhagic fever with renal syndrome epidemic in Shenyang City, China. BMC infectious diseases, 11(1), 331. London School of Hygiene & Tropical Medicine (2017, June) Where humans and animals collide: Emerging infections and newfound resistance. Retrieved from https://www.lshtm.ac.uk/research/research-action/features/where-humans-and-animals-collide-emerging-infections-and-newfound. Accessed March 11th, 2020. Lukan, M., Bullova, E., & Petko, B. (2010). Climate warming and tick-borne encephalitis, Slovakia. Emerging infectious diseases, 16(3), 524. McMichael, A. J. (2004). Environmental and social influences on emerging infectious diseases: past, present and future. Philosophical Transactions of the Royal Society of London. Series B: Biological Sciences, 359(1447), 1049-1058. Morse, S. S. (2001). Factors in the emergence of infectious diseases. In Plagues and politics (pp. 8-26). Palgrave Macmillan, London. Myhre, G., Alterskjær, K., Stjern, C. W., Hodnebrog, Ø., Marelle, L., Samset, B. H., ... & Stohl, A. (2019). Frequency of extreme precipitation increases extensively with event rareness under global warming. Scientific reports, 9(1), 1-10. Ogden, N. H., Radojevic, M., Wu, X., Duvvuri, V. R., Leighton, P. A., & Wu, J. (2014). Estimated effects of projected climate change on the basic reproductive number of the Lyme disease vector Ixodes scapularis. Environmental health perspectives, 122(6), 631-638. Okuthe, O. S., & Buyu, G. E. (2006). Prevalence and incidence of tick-borne diseases in smallholder farming systems in the western-Kenya highlands. Veterinary parasitology, 141(3-4), 307-312. Our World in Data (2019, May) World Population Growth. Retrieved from https://ourworldindata.org/world-population-growth. Accessed March 11th, 2020. Our World in Data (2019, November) HIV/AIDS. Retrieved from https://ourworldindata.org/hiv-aids. Accessed March 11th, 2020. Paaijmans, K. P., Blanford, S., Bell, A. S., Blanford, J. I., Read, A. F., & Thomas, M. B. (2010). Influence of climate on malaria transmission depends on daily temperature variation. Proceedings of the National Academy of Sciences, 107(34), 15135-15139. Payne, A. E., Jansen, M. F., & Cronin, T. W. (2015). Conceptual model analysis of the influence of temperature feedbacks on polar amplification. Geophysical Research Letters, 42(21), 9561-9570. Pecl, G. T., Araújo, M. B., Bell, J. D., Blanchard, J., Bonebrake, T. C., Chen, I. C., ... & Falconi, L. (2017). Biodiversity redistribution under climate change: Impacts on ecosystems and human well-being. Science, 355(6332), eaai9214. Pollard, D., DeConto, R. M., & Alley, R. B. (2015). Potential Antarctic Ice Sheet retreat driven by hydrofracturing and ice cliff failure. Earth and Planetary Science Letters, 412, 112-121. Qiu, W., Chu, C., Mao, A., & Wu, J. (2018). The Impacts on Health, Society, and Economy of SARS and H7N9 Outbreaks in China: A Case Comparison Study. Journal of environmental and public health, 2018. Thornton, J (2017, June) Where humans and animals collide: Emerging infections and newfound resistance. Retrieved from https://www.lshtm.ac.uk/research/research-action/features/where-humans-and-animals-collide-emerging-infections-and-newfound. Accessed March 11th, 2020. Tokarevich, N. K., Tronin, A. A., Blinova, O. V., Buzinov, R. V., Boltenkov, V. P., Yurasova, E. D., & Nurse, J. (2011). The impact of climate change on the expansion of Ixodes persulcatus habitat and the incidence of tick-borne encephalitis in the north of European Russia. Global Health Action, 4(1), 8448. U.S. Department of Defense (2014, October 13) Hagel to Address ‘Threat Multiplier’ of Climate Change. Retrieved from https://www.defense.gov/Explore/News/Article/Article/603440/. Accessed March 11th, 2020. Watson, R. T., Gitay, H., Suarez, A., Dokken, D. J., & Leemans, R. (2002). Climate change and biodiversity. WMO/UNEP: IPCC Technical paper V. Wiles, G. J., Lemke, T. O., & Payne, N. H. (1989). Population estimates of fruit bats (Pteropus mariannus) in the Mariana Islands. Conservation Biology, 3(1), 66-76. Wilhite, D. A. (2000). Drought as a natural hazard: concepts and definitions. World health organization (2020, March 11) WHO Director-General's opening remarks at the media briefing on COVID-19 - 11 March 2020. Retrieved from https://www.who.int/dg/speeches/detail/who-director-general-s-opening-remarks-at-the-media-briefing-on-covid-19---11-march-2020. Accessed March 16th, 2020.
Kim Nguyen
The risks of zoonotic diseases are rising in the Anthropocene partly attributed to climate change
Leonardo
Good afternoon,
My name is Leonardo and I work for the Ecorys consulting company in Brussels. I am currently working on behalf of the European Commission, DG ENV, as a project management assistant to the service contract Science For Environment Policy (SfEP). DG ENV is creating a brief on Covid-19 – as part of the Science for Environment Policy (SfEP) series – and some colleagues suggested that we may use some of your pictures / figures with no commercial purposes.
More precisely, we would be interested in re-using Figure 5. Data taken from Bean et al., (2013) on emergence of zoonosis (figure made by Ichiko Sugiyama) available at: https://blogs.egu.eu/divisions/cl/2020/03/16/corona/
We would like to kindly ask you the copyrights permissions to be able to reuse this figure(s) in our future brief which will be published by DG ENV shortly, if you are the ones entitled for this publication.
In case of re-use permission, would you be so kind to send us images in either JPEG or PNG format at 300ppi, which is industry standard for their applicability? We should try to avoid images below 200ppi as they start to look low quality in the document. Alternatively, we could explore some redrawing options for which we would still need to include copyright information.
Would you be kind enough to let us know at your earliest capacity if this request is possible and if you can kindly share with us the files as described above?
Thank you very much in advance for your nice collaboration.
My best regards,
Leonardo
Ichiko Sugiyama
Hello Leonardo,
I have sent you two documents yesterday, .ai and .png file, of the figure of interest. Please let me know if you require anything else and we are here to help. Thank you again for contacting us!