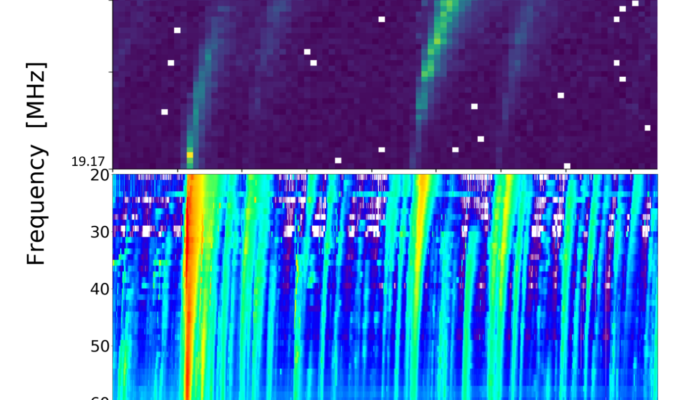
Large coronal loops around one solar radius in altitude are an important connection between the solar wind and the low solar corona. However, their plasma properties are not well studied, as standard X-ray and UV techniques are not suited to these low-density environments. How does temperature, pressure, and magnetic field strength evolve along these loops? Observable structures in radio emission that are of solar origin and are known as J-bursts are the signature of an accelerated electron beam traveling along coronal loops. Diagnostics from J-bursts at radio frequencies above 10 MHz are ideally suited to understand these coronal loops. They can also provide us with information necessary for understanding the kinetic properties of the electron beams travelling along these loops. For example, do they have the same velocities as the electron beam streaming out into the heliosphere along open magnetic flux tubes that generate type III solar radio bursts?
In our recently published work (Zhang et al., 2023), we studied electron beam velocities and physical parameters of large coronal loops using LOw-Frequency-ARray (LOFAR) J-burst observations during a solar storm recorded in radio frequencies.
The radio observations during a solar storm and how we classified type III and J-bursts
LOFAR Low-Band Array (LOFAR/LBA) observed the radio noise from a solar storm (known as the ‘solar radio noise storm’) between 20 to 80 MHz on 10 April 2019, which contains many J- and type III bursts. As the Parker Solar Probe (PSP) FIELDS instrument observed interplanetary (IP) type III solar radio bursts between 1.3 to 19.2 MHz simultaneously, we combined both dynamic spectra to build the full picture of radio burst measurements from 1.3 to 80 MHz, as shown in Figure 1. In this frequency range, the observed radio flux was generated by electron beams travelling from around 0.5 to 7 solar radii. Type III bursts show clear continuities between the two spectra and J-burst show a clear turnover frequency in the LOFAR dynamic spectrum before 20MHz. We identified 27 type-III bursts and 27 J-bursts associated to that solar eruption.
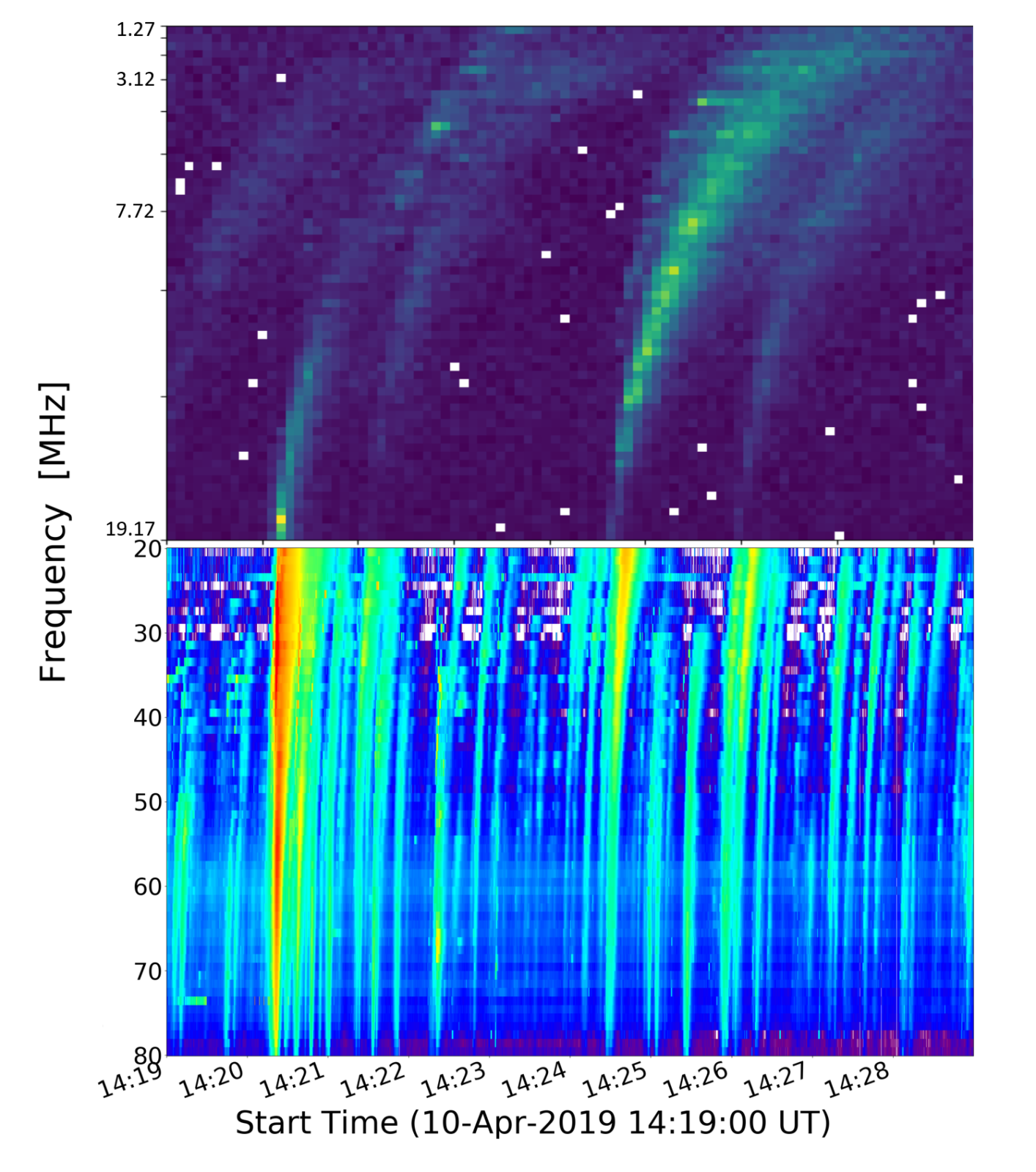
Figure 1. Ten minutes of data combining LOFAR and PSP dynamic spectrum, used for classifying type III and J-bursts during the noise storm on 10 April 2019. The top panel: PSP RFS radio observations between 1.3 – 19.2 MHz; The bottom panel: LOFAR LBA observation between 20 – 80 MHz. On this example dynamic spectrum, there are five interplanetary (IP) type III bursts identified in the PSP dynamic spectrum that are also observable by LOFAR. (Figure obtained by Zhang et al., 2023)
Electron beam velocities
Previous studies suggested that the velocities of electron beams producing J-bursts’ are similar to those producing type III bursts (e.g., Labrum & Stewart, 1970). However, it is not clear whether the same active region would produce accelerated electron beams with similar velocities near the foot-point of magnetic loops confined to the corona (emitting J-bursts) and magnetic loops that extend into the heliosphere (emitting type III bursts).
In our study, we used a classical method to determine the electron beam velocities from the frequency drift rate of the identified type III bursts they generated. The beam velocity (distance over time) can be calculated from the frequency drift rate (frequency over time), which was defined from the time profile of each maximum flux point from each frequency band as shown in Figure 2. However, this method cannot be directly applied on J-bursts. This is because the curved segment of the J-burst is associated to the curved features of the closed loops, where standard numerical coronal density models cannot be applied. We used the high frequency part of the J-bursts, which we named ‘type-III-like’ part of the J burst, to avoid the aforementioned problem, since this part has relevant constant high frequency drift rate and can be considered that the electron beam producing it travelled along ‘open’ field. We found that the exciters (electron beams) of these identified type III bursts have average velocity equal to 0.18 c, within one standard deviation of the average velocity of 0.22 c of the J-burst exciters. We do not see significant difference in the velocity of the electron beans that produced the J- and type III bursts. Thus, we suggest there is no discernible difference in the acceleration properties at the base of 1 solar radii loops, and the base of ‘open’ coronal magnetic field.
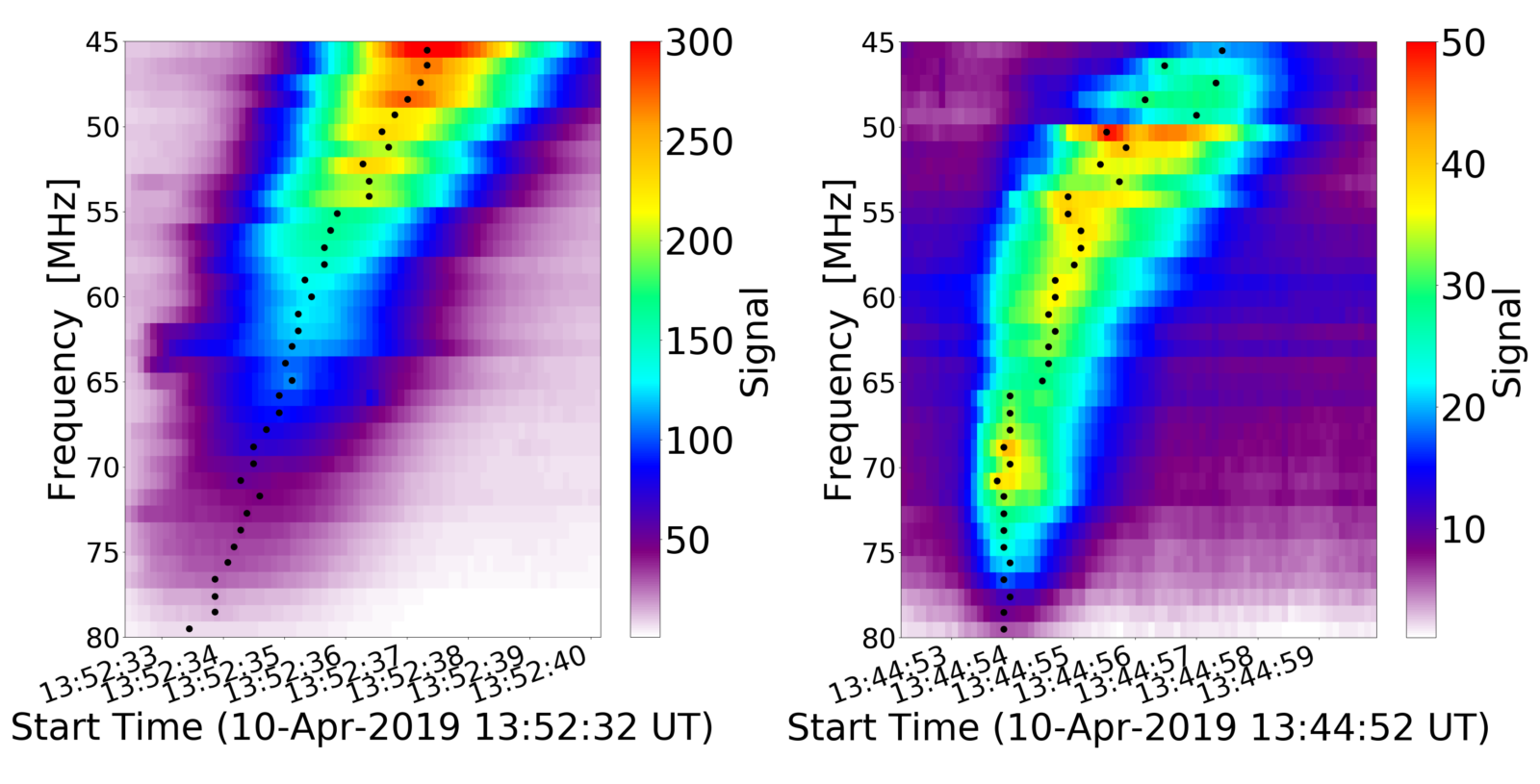
Figure 2. Type III burst (left) and a J-burst (right) on the dynamic spectrum. Type III and J-bursts show rapid drift from high to low frequencies. Type III bursts have relatively constant frequency drift rate, and J-bursts show decreased frequency drift rate. Black dots on both dynamic spectra are maximum-flux points for each frequency band, and their temporal profiles show frequency drift. For analysing the example J burst exciter, we use drift rate from the ‘type-III-like part’, which is between 65 – 80 MHz. (Figure obtained by Zhang et al., 2023)
Coronal loop physical parameters
To understand the physical characteristics of large coronal loops, we selected 24 J-bursts during the noise storm observed by LOFAR to determine the loop height, density scale height, loop top plasma temperature, pressure, and minimum magnetic field strengths. So far, these parameters are not well defined for these large coronal loops. To obtain estimates of loop parameters, we developed a method that overcame the problem of coronal density models not being applicable to the curved portion of J-bursts. This method is assuming that the electron beam velocity remains constant along the semi-circular loop-top shape. The mean physical parameters against the solar altitude were determined from 24 J-bursts as shown in Figure 3.
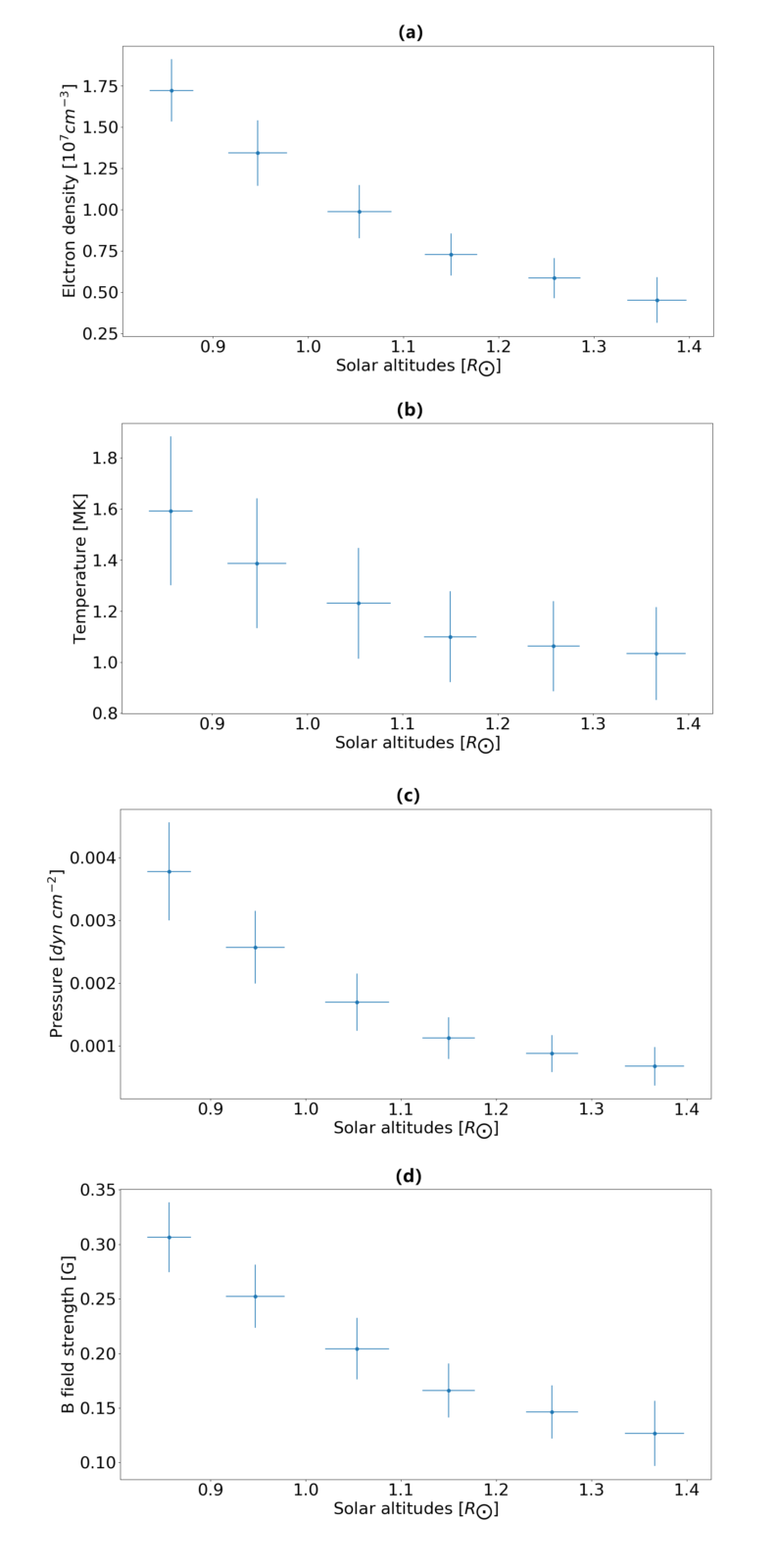
Figure 3. Coronal loop plasma parameters inferred from 24 J-bursts, averaged every 0.1 solar radius. (a) Electron density, (b) temperature, (c) pressure, (d) minimum magnetic field strength. Error bars are calculated from the standard deviations to show the spread in results. Each point in the figure is an average for each 0.1 solar radius. (Figure obtained by Zhang et al., 2023)
We found the average density scale height is 0.36 solar radius and the average loop apex altitude is around 1.37 solar radius. To infer the plasma temperature we used the definition of the coronal loop density scale height provided in Aschwanden et al. (1992). At the loop apex, we found an average plasma temperature of around 1MK. To determine the average plasma pressure at the loop apex, we applied the ideal gas law. We determined the minimum magnetic field strength by assuming that the plasma beta is less than 1 in the high solar corona. We thus estimated the plasma pressure at the apex of the coronal loop to be at around 0.7 mdyn cm−2. the average minimum magnetic field strength was then estimated to be approximately 0.13 G.
What’s next?
In our paper, we described methods for estimating electron beam velocities, inferring coronal loop density, loop-top temperature, pressure, and minimum magnetic field strength by using dynamic spectra data of J-bursts. We are currently working on a new project aiming in comparing our new methods with a density model based on interferometric images. The new generation radio interferometers such as LOFAR can provide high frequency and temporal resolution solar radio images. Employing observations from the new generation instruments, we can derive the radio source positions which can be further used for determining with higher accuracy the beam velocity and infer the coronal loop density model directly.
References
- Aschwanden M. J., Bastian T.S., Benz A. O., Brosius J. W., Decimetric Solar Type U Bursts: VLA and PHOENIX Observations, The Astrophysical Journal, 1992, doi: 10.1086/171353
- Labrum N. R., Stewart R. T., The Solar U-Burst at Metre Wavelengths, Proceedings of the Astronomical Society of Australia, 1970, doi: 10.1017/S132335800001208X
- Zhang J., Reid H. A. S., Krupar V., Zucca P., Dabrowski B., Krankowski A., Deriving Large Coronal Magnetic Loop Parameters Using LOFAR J Burst Observations, Solar Physics, 2023, doi: 10.1007/s11207-022-02096-0