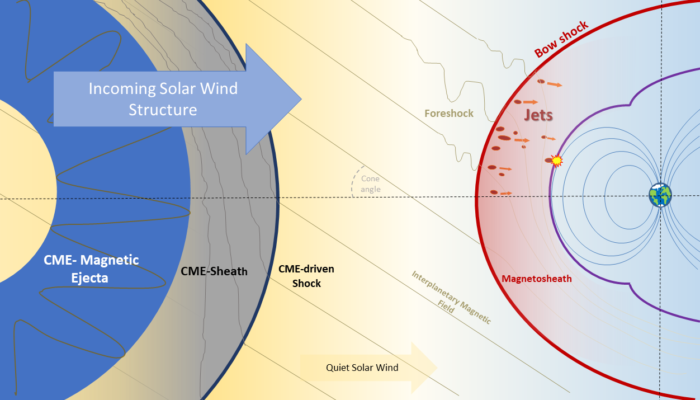
Dayside of the Earth’s magnetic field
Our understanding of the relationship between Earth’s magnetic field and the particles from the Sun that constantly bombard it, known as the solar wind, has significantly advanced in recent decades. The availability of numerous spacecraft measurements has provided valuable insights into this interaction. The solar wind, composed of high-speed particles carrying the interplanetary magnetic field from the Sun, undergoes a rapid deceleration as it collides with Earth’s magnetic field. This deceleration creates a shockwave, similar to a sonic boom, transforming the solar wind plasma into a slower, denser, and hotter state. This turbulent region, known as the magnetosheath, is formed at approximately 10-15 Earth radii sunward of our planet. Before entering the magnetosheath, any influence on Earth’s magnetic field must first pass through the bow shock, the initial shock encountered by incoming particles.
Extensive research on the magnetosheath has been conducted through spacecraft missions. In 1998, Němeček et al. reported an increase in particle flow within the magnetosheath that did not correspond to the prevailing solar wind conditions at the time. This phenomenon, characterized by heightened dynamic pressure with increased velocity and plasma density, is now known as magnetosheath jets.
Magnetosheath jets and their generation
Magnetosheath jets, which occur multiple times per hour (Plaschke et al. 2016), have been found to impact Earth’s magnetic field (see e.g. Hietala et al. 2018) and can be geoeffective (e.g. Norenius et al. 2021). Ongoing research suggests that the foreshock region, located upstream of the bow shock, plays a crucial role in jet generation. In this region, where the interplanetary magnetic field aligns parallel to the shock surface normal, particles can be accelerated back toward the Sun upon collision with the shock.
The resulting interaction between the opposing particle populations generates waves within the foreshock region, which are then convected back to the shock by the solar wind. This leads to various effects, including compressional structures in the foreshock and ripples in the surface of the bow shock. It has been theorized that the interaction between the solar wind and these ripples contributes to the formation of jets downstream of the shock (Hietala et al. 2013). Raptis et al. (2022) showed that jets can be caused by the reformation of the shock itself due to compressive magnetic structures in the foreshock. Global simulations further support the direct connection between foreshock compressional structures and the generation of jets. (Suni et al 2021). A minority of jets can be linked to other processes as well (see Plaschke et al. 2018 for a review), nonetheless, it has been shown that jets appear 9 times more likely in the magnetosheath region behind the foreshock and close to the bow shock (Vuorinen et al. 2019).
In any case, beneficial solar wind conditions are crucial for the creation and the survival of jets in the magnetosheath (see LaMoury et al. 2021). Different solar wind structures significantly alter these conditions, thus influencing the formation of magnetosheath jets. Coronal mass ejections (CMEs), large clouds of plasma (magnetic ejecta) expelled from the Sun with strong magnetic fields. CMEs can drive a shock ahead of them, forming a distinct CME-sheath region. On the other hand, stream interaction regions (SIRs) and high-speed streams, caused by fast solar wind from coronal holes, result in the compression of solar wind and the subsequent formation of a fast stream with lower density.
Understanding how these diverse solar wind structures impact the appearance of jets in the magnetosheath provides insights into the global energy flow connection from the upstream side to Earth’s magnetic field. Koller et al. (2022) showed that CMEs decrease the jet generation, while high-speed streams positively influence jet occurrence. A recent study by Koller et al. (2023) further explores the statistical influence of solar wind structures on the jet generation in greater detail.
Searching for the cause of modified jet occurrence
One of the most critical factors in understanding jet occurrence between Earth’s magnetic field and the shock is the cone angle. This angle represents the angle between the Sun-Earth line and the interplanetary magnetic field in the solar wind. A low cone angle indicates that the foreshock region is located closer to the Sun-Earth line, leading to the production of jets. On the other hand, a high cone angle positions the foreshock region closer to the flanks, resulting in fewer jets in the subsolar region. Another significant parameter is the Mach number, which represents the ratio of the solar wind speed to the local speed of information within the medium. Shocks with a high Mach number accelerate particles back towards the Sun, thus causing the foreshock. A low Mach number weakens the shock, resulting in a less efficient build-up of the foreshock region. Therefore, these two parameters, cone angle and Mach number, play a crucial role in jet generation.
In Figure 1a, the distribution of cone angles and Alfvén Mach number in the solar wind is analyzed and compared to the distribution of the solar wind during the detection of jets (Figure 1b). Figure 1c shows the occurrence rate for each solar wind condition (resulting from dividing the previous two distributions). Figure 1d shows the corresponding errors. The analysis reveals that a low cone angle is favorable for jet generation as expected. High cone angles and low Alfvén Mach numbers show the lowest likelihood of jet occurrence.
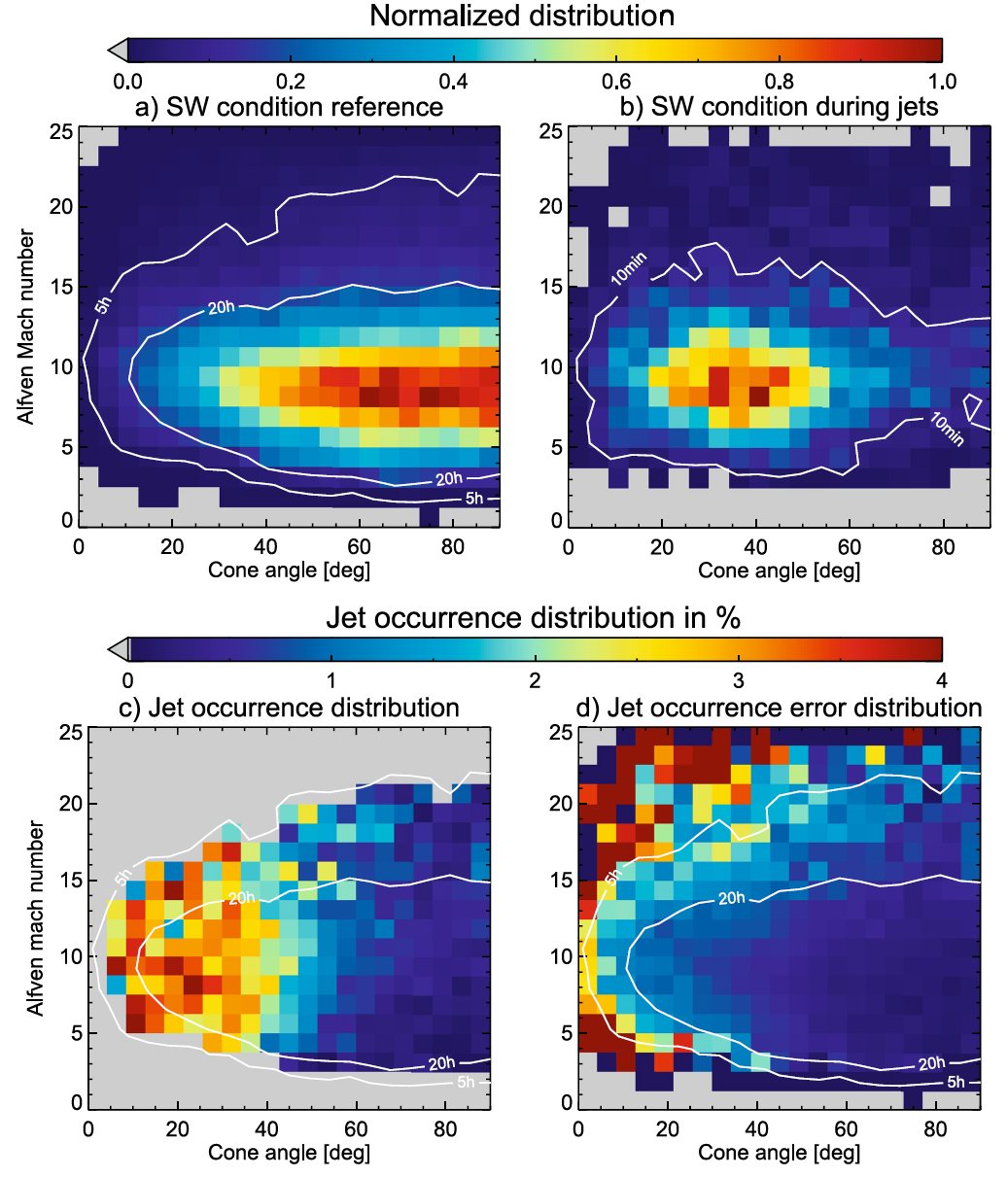
Figure 1: 2D histogram showing distributions of cone angle and Alfven Mach number. Plot (a) shows the overall distribution of both parameter in the solar wind during all observation times, plot (b) shows the SW parameter distribution during jet detection. Plot (c) shows the jet probability depending on both parameters, plot (d) shows the corresponding errors.
Next, let’s examine how the distribution looks for each solar wind structure (Figure 2). It is observed that the distribution for the magnetic ejecta of CMEs (Figure 2b) and their sheath (Figure 2a) peaks at low Mach numbers and high cone angles, coinciding with the parameter distribution associated with the lowest probability of jet occurrence. The SIR and HSS distributions show a range that more frequently overlaps with higher jet probability.
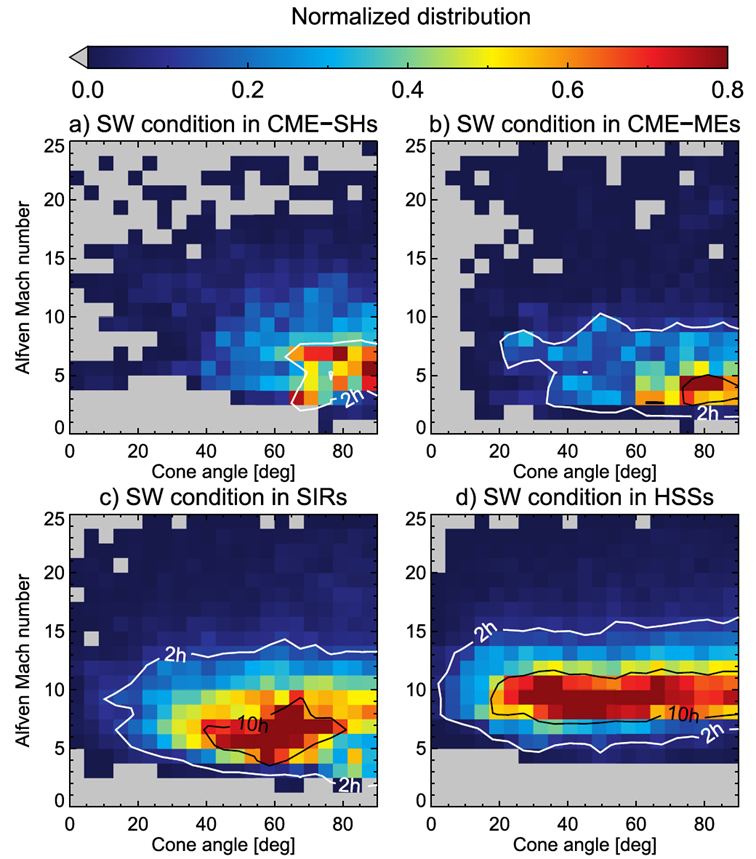
Figure 2. 2D histogram showing distributions of cone angle and Alfven Mach number for each type of solar wind structure: (a) during coronal mass ejection (CME)-sheaths, (b) during CME-magnetic ejectas (MEs), (c) during stream interaction regions SIRs) and (d) during high-speed streams (HSSs). Contours indicate the number of available hours of data.
Koller et al. (2023) extended the analysis to all parameters to see how the jet occurrence distribution is related to each individual parameter. The jet occurrence is then compared to the distribution of all parameters in both CMEs and SIRs. The statistical distribution of most parameters in CMEs indicates overall unfavorable conditions for jet generation. The diminished Mach number is likely a result of the high magnetic field strength within the magnetic ejecta of CMEs. On the other hand, high-speed streams exhibit ideal conditions for jet generation (agreeing with the findings of LaMoury et al. (2021)), which include high velocity, often low cone angles, low magnetic field strength, low density, high Mach numbers, and high plasma beta.
Conclusions and implications of our work
CMEs and CME-sheaths have a negative impact on jet occurrence due to diminished foreshock building, while the compression region of SIRs has a neutral effect. On the other hand, high-speed streams have a positive influence on jet occurrence due to their potential to cause a foreshock region near the Sun-Earth-line. This analysis also provides an overview of the statistical parameter distributions for each type of solar wind structure measured at Earth.
It is worth noting that jet occurrence drastically drops for very low Mach numbers. Although this condition is rare in the solar wind, it is relatively common inside CMEs. This finding has implications for other magnetosheaths as well, such as at Mercury, where low Mach numbers are more common This suggests a potential decrease in jet generation. Measurements by BepiColombo should provide further clarity on this matter in the future.
References
H. Hietala, et al. 2018, Geophysics Research Letters, 45(4), 1732–1740. (https://doi.org/10.1002/2017GL076525)
H. Hietala, and F. Plaschke, 2013, Journal of Geophysical Research: Space Physics, 118(11), 7237–7245. (https://doi.org/10.1002/2013JA019172)
F. Koller, et al. 2022, Journal of Geophysical Research: Space Physics, 127(4), (https://doi.org/10.1029/2021JA030124)
F. Koller, et al. 2023, Journal of Geophysical Research: Space Physics, 128(3), (https://doi.org/10.1029/2023JA031339)
A. T. LaMoury, et al. 2021, Journal of Geophysical Research: Space Physics, 126(9), (https://doi.org/10.1029/2021JA029592)
Z. Němeček, et al. 1998, Geophysics Research Letters, 25(8), 1273–1276. (https://doi.org/10.1029/98GL50873)
L. Norenius, et al. (2021, Journal of Geophysical Research: Space Physics, 126(8), (https://doi.org/10.1029/2021JA029115)
F. Plaschke, et al. 2016, Journal of Geophysical Research: Space Physics, 121(4), 3240–3253. (https://doi.org/10.1002/2016JA022534)
F. Plaschke, et al. 2018, Space Science Reviews, 214(5), 81. (https://doi.org/10.1007/s11214-018-0516-3)
S. Raptis, et al. 2022, Nature Communications, 13(1), 598. (https://doi.org/10.1038/s41467-022-28110-4)
J. Suni, et al. 2021, Geophysical Research Letters, 48(20), (https://doi.org/10.1029/2021GL095655)
L. Vuorinen, et al. 2019, Annales Geophysicae, 37(4),689–697. (https://doi.org/10.5194/angeo-37-689-2019)