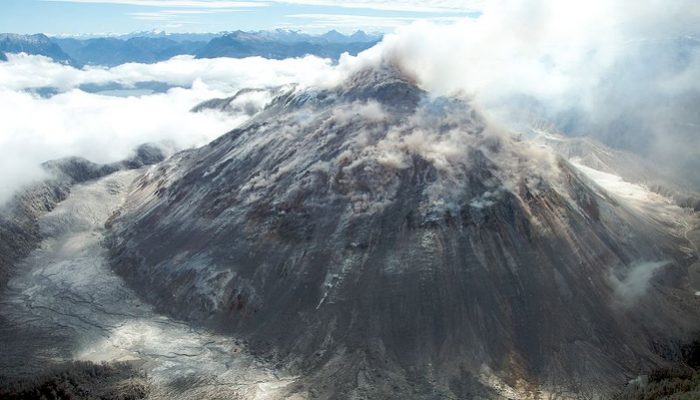
Lava domes form when lava is extruded from a volcanic vent, but is too viscous to flow far away. Think of thick treacle that does not flow as easily as runny honey, and so when it is extruded, it forms a “lava pile” around the vent. Lava domes commonly form within the crater of a larger volcano (e.g. Mt. St. Helens), but can also stand alone or form part of a “dome complex”.
A lava dome can take on a variety of shapes and sizes dependent upon many factors, including lava chemical composition, viscosity and extrusion rate. Some of the different types of lava domes range from “pancake domes”, generally short and wide, to “spiny domes”, where lava cools and breaks into blocks of rock. Volcanologists have observed over time that every lava dome is different, even at the same volcano or during the same eruption. The number of factors that influence the behaviour of a lava dome makes it difficult to understand the processes occurring during lava dome growth. There is still a lot of research necessary before we can think about forecasting behaviour during a dome-building eruption.
Lava dome hazards
There are many hazards associated with dome-building eruptions, and they are often linked to explosive volcanic activity. In the same way that landslides occur in a slope made of soil, lava domes can collapse if they become oversteepened. Lava dome stability can also be affected by intense bouts of rainfall, as the rainwater can be pressurised when it comes into contact with the hot dome (temperatures at eruption are generally in excess of 850˚C), thus turning the rainwater into steam and forcing the solidified lava to fracture apart, causing collapse. Other causes for collapse include a build-up of gas within the dome, or a change in direction of the lava extrusion.
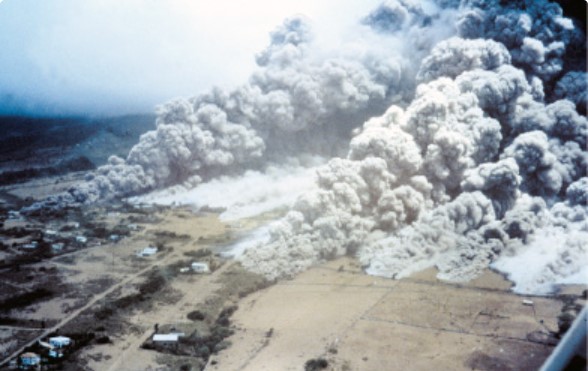
Pyroclastic flows from dome collapse in Montserrat, June 1997. Credit BGS.
A number of things can happen when a lava dome collapses, ranging from small scale rockfalls, to debris avalanches, to pyroclastic density currents (turbulent mixtures of gas and rock that can travel at speeds of over 100 kilometres per hour). Any of these can be devastating to communities surrounding the volcano, as pyroclastic density currents cannot be redirected using the same methods that have been utilised for lava flow diversion.
Hazards for scientists
Despite how dangerous these events can be to populated areas, very little is known about various causes of a collapse. This is mainly because the internal structure of a lava dome is uncertain. We think there is a ductile, frictionless core that is surrounded by a solid, friction-controlled rind (think of a soft caramel encased by hard chocolate). A dome often also includes talus aprons, which are piles of loose rubbly material that accumulate due to rockfalls that occur during dome growth.
Research into lava dome collapse can be split into three main areas: field observations, analogue and laboratory experiments, and numerical modelling. Field observations of real life environments and landscapes are vital for creating conceptual models of a lava dome.
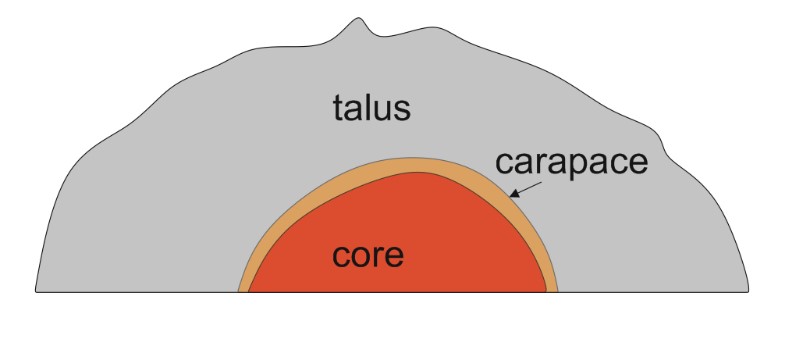
Conceptual diagram of internal structure, and photo of the dome at Mt. Unzen (2016). Credit: Claire Harnett
The missing particle of the puzzle
After fieldwork at both Mt. Unzen and Soufrière Hills Volcano, my research has focused on numerical modelling of lava dome collapse. The aim is to build a computer simulation of a lava dome, and then change conditions in the model to create various “model scenarios” for collapse. This allows us, for example, to change the gas pressure (something we know to cause collapse at a real dome) in our synthetic lava dome, and vary the time period over which forces are exerted upon the dome.
To do this, we use discrete element method (DEM) modelling. DEM considers the simulated lava dome as an assembly of individual particles/blocks, which means that the model can evolve dynamically and the particles act according to gravity and their interactions with neighbouring particles. We generate a particle based material that represents lava. We then give the particles a speed and a direction to simulate extrusion, and we control the point at which parts of the material start to behave as a solid (i.e. representing a realistic cooling/solidification process).
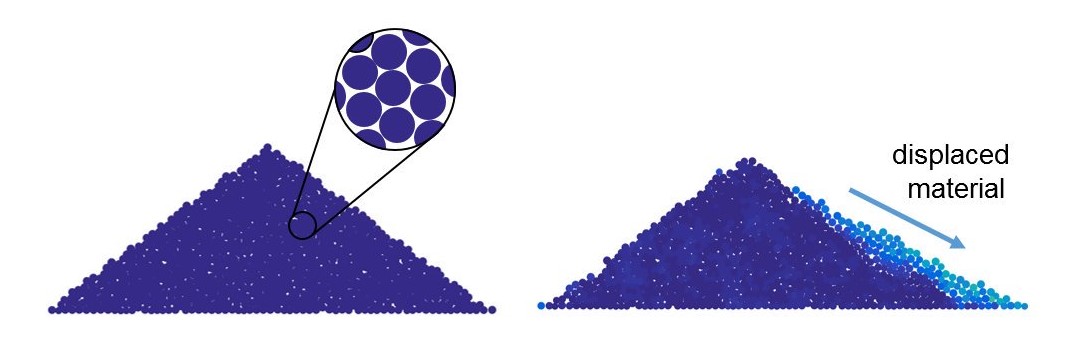
Discrete element model of a very simplistic lava dome. Credit: Claire Harnett
Although these models are still in development, we are able to replicate the growth patterns observed at lava domes. For example we see when a lava dome reaches its maximum height (determined by the rock properties), it starts to grow outwards rather than upwards. We aim to create software that can further our understanding of the processes occurring within a dome, with the ultimate goal of use in observatories. Scientists monitoring the volcano could then input what they see occurring during a volcanic eruption, and have a greater chance of predicting what will happen next.
These models are proving to be a really exciting and innovative way to study lava dome growth and collapse, and will reveal some of the mysteries of lava domes and the dangers they pose.
A movie of lava dome emplacement, where red material acts as ductile core, and grey material acts as solid rock.
Blog post written by Claire Harnett. For more information, keep your eyes peeled for the paper coming soon! Or get in touch with Claire on Twitter.