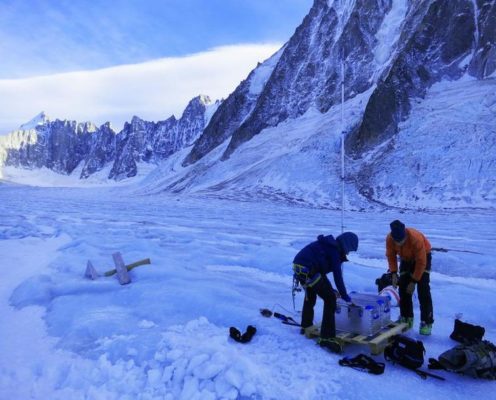
Have you ever wondered what the voice of a glacier sounds like? Well, listen here!
And if you want to know how the glacier makes these sounds, then let’s take a walk on the ice side…
Close your eyes and think of the time you were in the middle of the mountains. On a snow plain, a glacier or a frozen lake; just you. You hear your footsteps in the snow, crunching ice. The wind blows through your hood. And the silence. A pristine silence, without smell. A dull silence, like in cotton. Now stop in the snow. You feel the cold, the wind stops. And as you listen, you begin to hear noises so slight, you think you are dreaming. These noises come from the heart of the glacier, from its surface and its core, and betray its sly movement. A sleeping ice dragon that dreams and stirs. Glaciers, ice flows, polar ice caps or ice shelves do not remain silent and the slightest movement generates a vibration that for attentive ears such as those of scientists, holds crucial information about the dynamics of these glaciers. These scientists are cryoseismologists. Their job is to analyse the vibrations produced by glaciers to understand how they move, how they react to surface melting, how crevasses open up or what happens under hundreds of meters below the ice. The vibrations they study are often so weak, and at such low frequencies that they cannot be heard by the human ear. To record these vibrations, these scientists use seismometers, devices traditionally used to study earthquakes. So now we’ve come to the end of our walk, it’s time to talk science!
What is a seismic signal?
The vibrations recorded by a seismometer are known as seismic signals. You know, those black lines that used to be drawn on paper with a waving stylus? A seismic signal is characterized by its power (the amplitude of the signal) and its frequency (the number of vibrations per second expressed in Hz). As with sound, low frequency signals can travel much further in space than high frequency signals. This is why you can hear the bass of a song from much further away than the lyrics. The difference between a sound signal and a seismic signal is only its frequency. The sounds we hear in everyday life typically have a frequency between 20 and 20 000 Hz. But the seismic signals used in geophysics are usually below 100 Hz.
Traditionally, seismology has been mainly used to study the Earth’s interior at very low frequencies (< 0.1 Hz; less than one vibration every 10 seconds) using the vibrations generated from earthquakes or volcanic eruption. It has been used to reveal the structure of the Earth (e.g., crust, mantle, chemical compositions), to locate mineral or oil deposits, and to obtain information on earthquakes (e.g., mechanisms, slip motion, location of the epicenter).
A more recent use of seismology is the study of the Earth’s surface at relatively high frequencies (>1 Hz; more than one vibration per second). This involves the study of vibrations generated by surface and sub-surface processes influenced by environmental parameters (e.g., weather conditions, hydrology, human activity). In contrast to traditional methods, environmental sources often exhibit noisy signals, such as the sound of flowing water, due to the complexity of the subsurface environment (e.g., stratification, unconsolidated sediments, water content) rather than clear short-lived pulse (e.g., the sounds of ice cracking) (Figure 2). As short-lived pulses are easily identifiable on a seismogram, they were deemed more useful than the other part of the signal, leading to the signal from environmental sources to be referred to as “noise”.
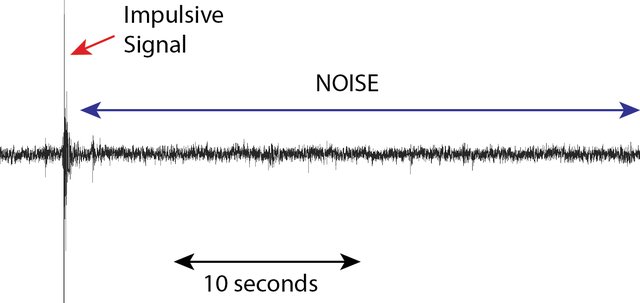
Figure 2: Seismic signal showing an impulsive source, generated from a crevasse opening, and noise signal, generated from flowing water at the surface of an Alpine glacier. Credits: Ugo Nanni
However, in the last two decades, advances in seismological technology have opened up a wide range of possibilities for using this noisy signal, such as monitoring and studying the physical properties of mass movements (landslides, rock falls), water flow (groundwater, open water, ocean currents), erosion (sediment transport, abrasion), human activity (traffic noise) and natural hazards (volcanoes, tornadoes). But most importantly for us, it has allowed the development of cryoseismology, using noise to monitor avalanches, icequakes, fracturing and thawing permafrost.
Seismic Signals Produced by the Cryosphere
If you have ever been near a glacier calving, crevasse opening, ice avalanche or supraglacial rivers, you may have noticed that all these processes generate different sounds, and therefore produce seismic waves. The magnitude of most cryoseismic events that occur is often thousands of millions times smaller than that of a magnitude 5 earthquake. Each of these mechanisms for producing seismic waves can be used in a different way, let me tell you more!
Surface crevassing
Crevasses, i.e. wide fractures in the ice, are what we are scared of when walking on a glacier. A direct pathway to the glacier’s interior. They are visible manifestations of the stresses within the ice and are observed on any kind of glaciers, even on Mars! But they are problematic to study, as they are difficult to access, (well, actually, they are very easy to access if you fall in, but very difficult to exit!), and they are often hidden under snow. However, just like the ice fractures, small vibrations are created when the ice breaks, and they propagate over long distances (up to hundreds of kilometers for a very large rupture), where they can be recorded by a seismometer, installed in a safe area. The study of crevasse seismicity has contributed significantly to the understanding of the forces acting within a glacier, which is of great importance for understanding ice streams (very fast moving glaciers) or tidally modulated glaciers (marine-terminating glaciers influenced by tides) but also for assessing glacier hazards.
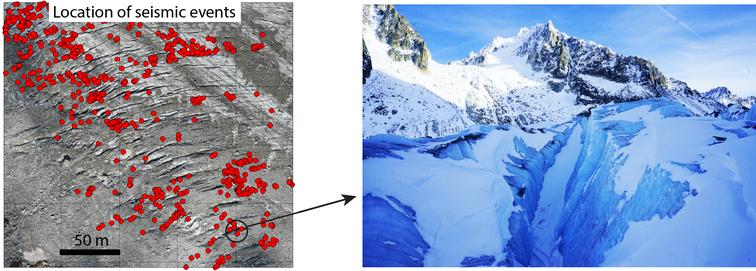
Figure 3: Example of seismic events location (red dots) at the surface of the Argentiere glacier together with a field picture of one of the crevasse. The picture on the left panel was taken by drone by Bruno Jourdain, the one on the right by Benoit Urruty. Check here for details on this research.
Subglacial friction:
Glacier stability depends largely on the ice-interface contact, where the base of the glacier slides over rocks and sediments (Figure 4). Glacier dynamics are traditionally described by viscous deformation, but field observations and laboratory experiments of seismic events originating at the base of the glacier have challenged this description. They have observed short-lived events that can only be caused by brittle deformation of the glacier, which may require reconsideration of the traditional description. Several studies have shown that these events can also be used to monitor glacier stability, as seismic activity tends to increase before ice avalanches. Such observations, especially those obtained from laboratory experiments, are also used as analogues to better understand the physics of earthquakes.
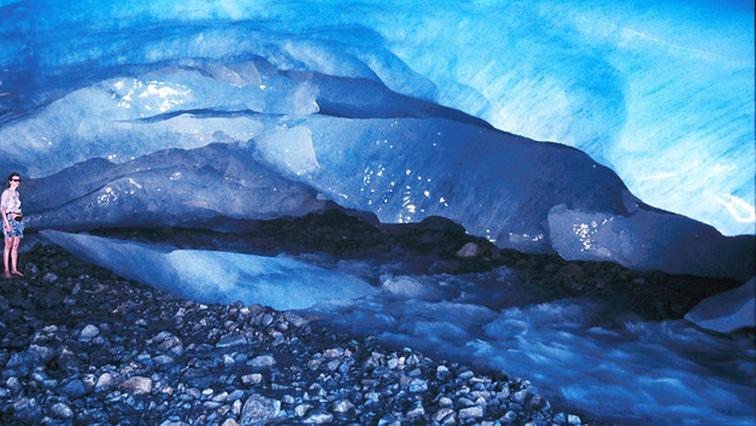
Figure 4: Water and rocks under an Alpine glacier. The movement of the glacier over such rough bed create small vibrations as ice slide over the rocks. Credits: Banco de Imágenes Geológicas.
Making icebergs: calving events
The release of icebergs from marine-terminating glaciers (calving, Figure 5) contributes to the frontal retreat of polar glaciers and ice caps and is responsible for most of the mass loss in Greenland and Antarctica. The volume loss produced during a calving event ranges from the size of an ice crystal to more than a trillion cubic meters. These events can last for several minutes and are similar in magnitude to a magnitude 2-3 earthquake. Detection and modelling of these events using seismology has increased over the last decade, leading to a better understanding of the physics involved in predicting future changes in mass loss over Greenland and Antarctica.
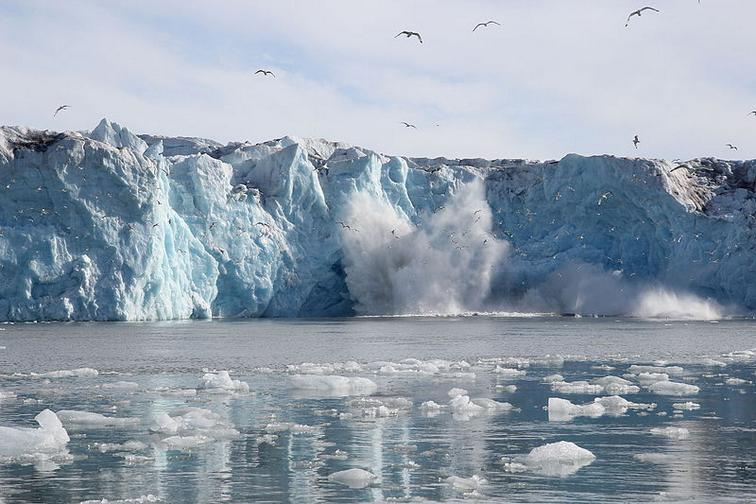
Figure 5: A calving event at the front of Monacobreen in Svalbard. From this image, it is quite clear how calving events can generate huge vibrations. Credit: Wikimedia Commons.
The sounds of water: glacier hydraulics
Meltwater produced at the surface and flowing to the base of glaciers plays a key role in glacier dynamics as it lubricates the ice-bed interface (Figure 4). However, the subglacial environment is difficult to access, because … it is a subglacial environment with hundreds of meters of ice on top! But turbulent water generates vibrations (and therefore seismic signals) that propagate up to the glacier surface. In contrast to the examples shown above that have been studied for over 50 years, the noise of water has been studied for less than 10 years.
This new area of cryoseismology is what I research! When we monitored subglacial water flow induced seismic noise for two years in the Alps (Figure 1), we observed clear seasonal variation in the seismic power recorded at the surface of glaciers (red curve in Figure 6). We observed that within a given frequency range (here [3-7] Hz), the seismic power was closely related to the variations in meltwater production caused by warm summer temperatures, providing a link between weather conditions, meltwater production and seismic noise. Similar studies are now conducted all over the world, from the Himalayas to the Antarctic and Arctic, allowing an estimation of the total amount of water transiting under glaciers to be calculated, and its impact on the glacier dynamics.
And many more:
You are almost reaching the end of the story, and you have only started to scratch the surface of the ice! I have introduced to you the most common sources of seismic activity in glaciology, but many more exist. I have not told you about the daily breathing of a glacier; as temperature changes during the day the cracks of the ice change as well. I have not told you about the song of the snow as it compacts, nor have I told you about the musical show of sea-ice as it dances with the wind. I leave you with some reading if you want to explore the vibrations of glaciers further.
Further Reading and hearing
- A very nice blog post that explain how to measure the energy of earthquakes
- A little history about cryoseismology
- Podolskiy & Walter (2016). Cryoseismology. Reviews of geophysics 54:708-758.
- The PhD thesis of the blog’s author about cryoseismology. You can find a more detailed introduction about the current challenges and future perspectives in this field: Resolving subglacial hydrology network dynamics through seismic observations on an Alpine glacier, 2020.
- An amazing calving event captured by the “Chasing Ice” team
- Cuffey & Paterson (2010) The physics of glaciers. Academic Press.
- Volcanoes do similar sounds than glaciers
- Biosphere, a norwegian artist who uses polar sounds to compose his music
Edited by Emma Pearce and Giovanni Baccolo
Ugo Nanni is a postdoctoral researcher at the University of Oslo. He uses cryoseismology to investigate the properties of the subglacial environment and understand what controls the stability of glaciers. He recently left the French Alps for Norway to listen to the murmur of the Arctic glaciers. Feel free to contact him for any questions: ugo.nanni@geo.uio.no, researchgate profile.
Irwansyah
nice mountain