When the Antarctic ice sheet loses mass, the pressure it exerts on the underlying solid Earth decreases. As the ice sheet becomes less heavy, the Earth’s surface is not pressed down as much as before and therefore slowly rises up. In some regions, this rebound process is much faster than previously thought and could stabilise areas of unstable ice retreat. How come? Keep reading to figure it out…
PS: You’ll find some clues in the picture!
What is Glacial Isostatic Adjustment?
The solid Earth beneath our feet is actually way softer than you might think and changes shape in response to changes of loading at its surface. This happens everywhere and all the time: the planet’s solid surface (called the lithosphere) is constantly adapting to the pressure exerted on it. The resulting vertical movements of the lithosphere can be very rapid, in response to tides for example. They can also be slower, in response to changes in heavier loads, such as the ice sheets. The latter, slower, change is what we call Glacial Isostatic Adjustment (often simply called GIA). You might remember this previous blog post, in which we explained that the Earth’s surface was currently still changing shape in response to the melting of the Northern Hemisphere ice sheets, that took place several thousands of years ago at the end of the last ice age. This demonstrates how slow that process can be!
How does it work?
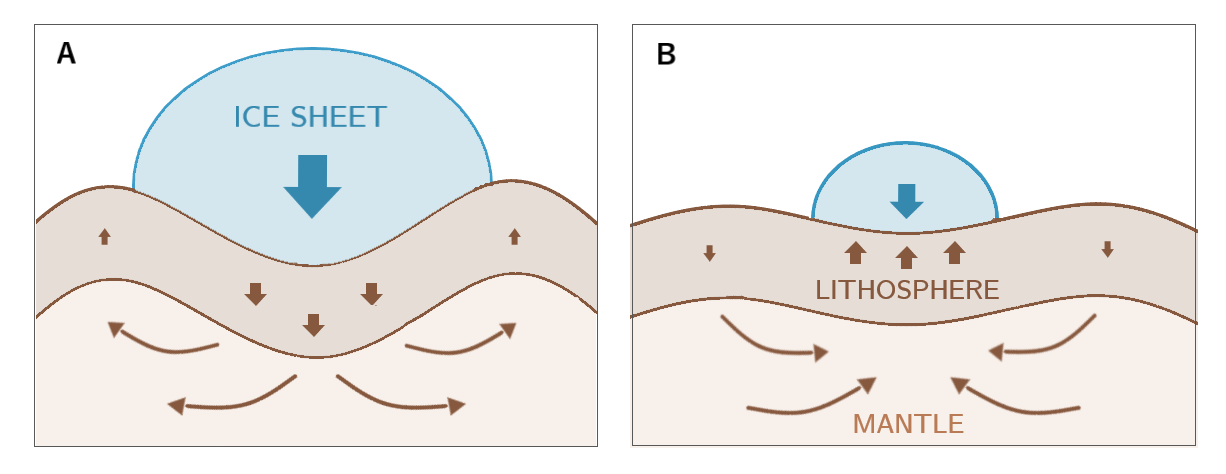
Figure 1: Solid Earth subsidence (A) and rebound (B) due to ice loading. The Earth mantle lying beneath the lithosphere behaves as a viscous fluid, responding to changes in ice sheet loading [Credit: Violaine Coulon].
An ice mass as huge as the Antarctic ice sheet (if it were to melt completely, sea level would rise by about 57 meters!) exerts a very important pressure on the underlying continent. As a consequence, the lithosphere has sinked in quite deep into the Earth’s viscous (meaning that it does not flow easily) mantle (Figure 1A). When the ice sheet melts, the pressure that it once exerted on the solid Earth is released. In order to counterbalance that loss of loading, the lithosphere rebounds (Figure 1B)!
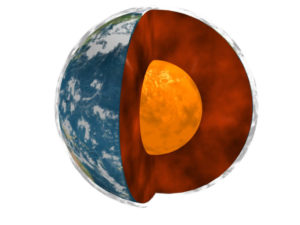
Figure 2: The Earth’s internal structure, layeres in spherical shells: the outer solidlithosphere, the viscous mantle, and the core [Credit: NASA/JPL-Université Paris Diderot – Institut de Physique du Globe de Paris].
To better understand how this works, imagine sitting on an exercise ball, except that it is not filled with air, but with honey. The ball would represent the Earth, with its surface representing the Earth’s lithosphere and the thick sticky honey representing the inside of the Earth: the viscous mantle (see Figure 2). As for you, you represent a heavy ice sheet, the weight of your body changing the shape of the surface of the ball, making it sink into the underlying honey. Once you get up (representing a melting ice sheet), the ball’s surface will straight away rebound a little bit. This is what is called the elastic rebound and is the immediate response of the solid lithosphere to the loss of pressure. But the surface of the ball will actually take quite some time to rebound to its original shape. This is because of the honey lying beneath the surface of the balloon. The viscous honey was pushed aside when you pushed on the ball with your body, and now, because of its high viscosity, it needs some time to flow back to its initial configuration. This is the viscous response.
Well, the same thing is happening — at a different scale — with an ice sheet exerting pressure upon the solid Earth. The time it will take to rebound actually depends on the viscosity of the Earth mantle (or of the honey in our ball example): the less viscous the mantle, the faster the Earth will react to load changes!
The unstable Antarctic Ice sheet
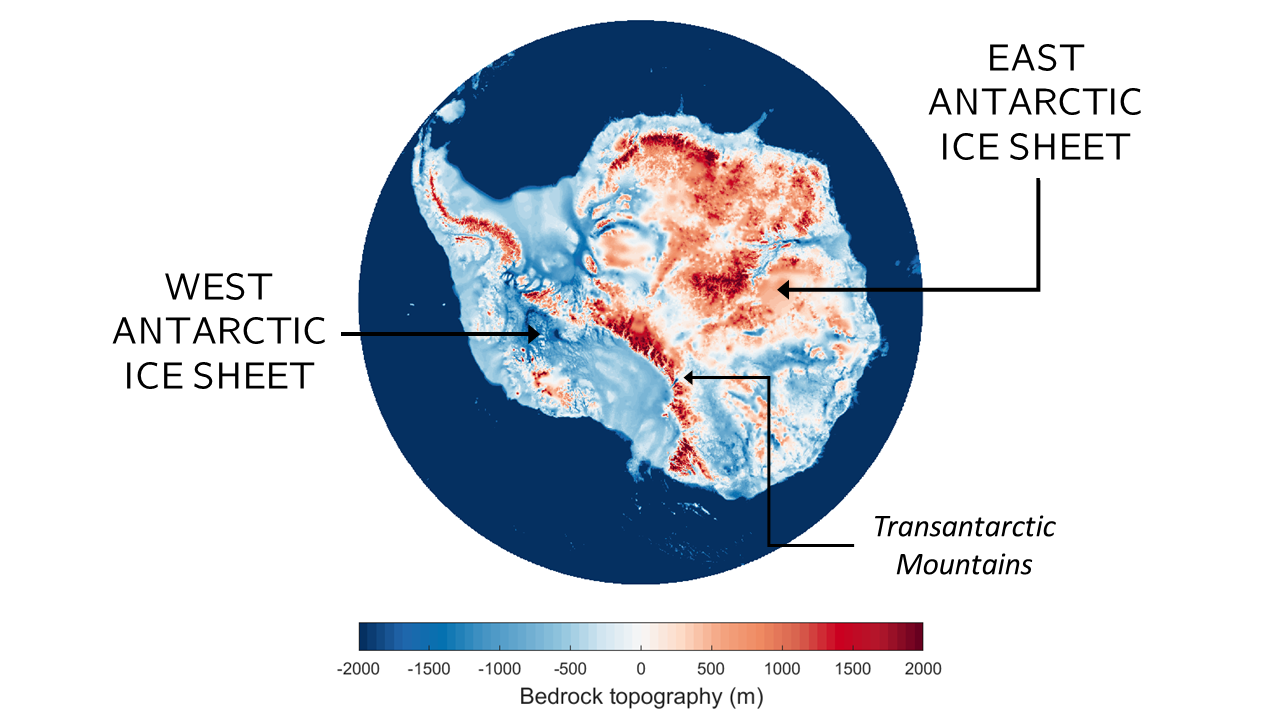
Figure 3: The Antarctic bedrock, largely lying below sea-level (blue areas). The Transantarctic Mountains divide the West Antarctic ice sheet from the East Antarctic ice sheet [Data Credit: Bedmap2, BAS; Figure by Violaine Coulon].
When observing the bedrock on which the Antarctic ice sheet is resting (Figure 3 above), you might notice that a big part of the continent is actually lying below sea level. This is mainly the case for the West Antarctic ice sheet, but also for some basins of the East Antarctic ice sheet. Because of the particular submarine location of their bedrock, these areas are called marine ice sheets. In addition, these same regions are very often characterized by an inward deepening bedrock, meaning that it gets deeper towards the interior of the continent. You can observe this on Figure 1, or on some great visualisations here.
The combination of these two characteristics makes the marine ice sheets sensitive to a specific instability mechanism, called the Marine Ice Sheet Instability (or MISI, check out this post for a more in-depth explanation). This instability is based on the following fundamental observation: the amount of ice that flows from the ice sheet towards the ocean (and thereby contributes to sea-level rise!) depends on how thick the ice sheet is at the position at which it starts to float on the ocean (called the grounding line). The thicker the ice sheet is at the grounding line, the larger is the amount of ice that is transferred from the ice sheet to the ocean.
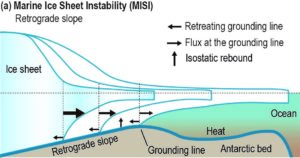
Figure 4: Schematic representation of Marine Ice Sheet Instability (MISI) from Pattyn (2018). Because bedrock under the ice sheet is sloping towards ice sheet interior, thinning of the ice causes retreat of the grounding line followed by an increase of the seaward ice flux, further thinning of the ice margin, and further retreat of the grounding line. [Credit: IPCC, SROCC]
When a marine ice sheet loses mass and shrinks, its grounding line retreats further inland, meaning, in the case of an inward deepening bedrock, in deeper bedrock areas (see Figure 4). As a consequence, the ice thickness at the grounding line will be larger, leading to even more mass loss, and thus a re-enforced retreat, and so on: it is a vicious circle that can lead to a runaway ice loss unless the grounding line reaches an area where the bedrock rises upward.
Because of its sensitivity to that marine ice sheet instability, the West Antarctic ice sheet has the potential to significantly contribute to future sea-level rise! Since most of its bedrock is lying well below sea level, if such an instability is triggered, the ice sheet could lose very rapidly most of its ice — we say that it would collapse. Today, it appears that some glaciers such as the Thwaites and Pine Island glaciers in West Antarctica, which are currently responsible for the largest contribution of Antarctica to global sea level rise, might already be undergoing a marine ice sheet instability… But the good news is that some processes are able to counteract and halt that instability (see for example this post), and glacial isostatic adjustment is one of them!
How can the isostatic rebound stabilise a melting ice sheet?
To understand how GIA is able to stabilise a marine ice sheet undergoing unstable retreat, it is important to understand the following concept: whether a given thickness of ice floats or not on the ocean depends on the depth of the ocean (meaning the difference between the sea surface and the bedrock if we removed the ice, see d1 on Figure 5a below). This is Archimede’s principle of buoyancy. Imagine an ice cube floating inside a glass of water. If you drink enough of that water, the ice cube will, at some point, stop floating and rest on the bottom of the glass. However, if you let it melt for some time so that it gets thinner, it will start to float again! Well, the same thing is happening with an ice sheet in the ocean. The grounding line is located where the ice becomes thin enough to float on the ocean. If the ocean depth decreases (for example if bedrock rises or if sea level decreases), some of the ice that was once floating will now rest upon the underlying bedrock (we say that the ice is grounded). As a consequence, the grounding line moves towards a shallower position, where the ice is thinner.
This is exactly what happens with glacial isostatic adjustment! When the grounding line of a marine ice sheet retreats further inland, the bedrock will react to the loss of grounded ice: it will start to rebound! And if the bedrock rebounds, it means that the water depth will be reduced: the grounding line will re-advance (Figure 5)! Isostatic rebound is thus able to counteract the marine ice sheet instability mechanisms.
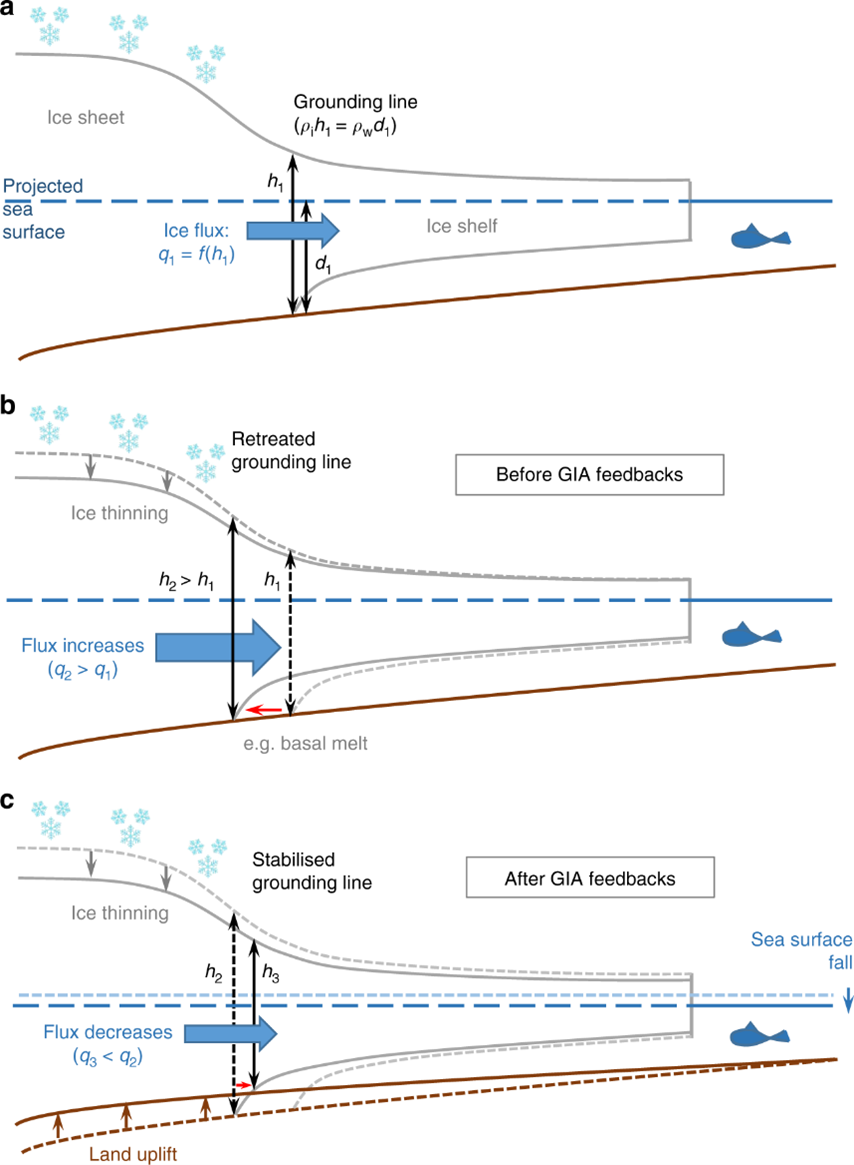
Figure 5: The stabilising effect of glacial isostatic adjustment (GIA) on ice dynamics. On a reverse bed slope, grounding line retreat leads to runaway ice loss due to the Marine Ice Sheet Instability. However, following grounding line retreat, the loss of grounded ice triggers land uplift. The resulting decrease in water depth (difference between the sea-surface and the bedrock if we removed the ice sheet) and hence ice thickness at the grounding line results in a decrease in ice flux across the grounding line, which acts to stabilise the ice sheet.
[Credit: Whitehouse et al. (2019), Nature Communications]
Therefore, it is very important to understand how the bedrock underneath the Antarctic ice sheet is going to react to further melting. The faster the solid Earth rebounds in response to ice mass loss, the faster the water depth will be reduced, and the easier a marine ice sheet may be stabilised. It is thus very important to better know the configuration of the solid Earth beneath the Antarctic continent, and more specifically, to answer the following question: how viscous is the Earth mantle?
So, how viscous is the mantle beneath Antarctica?
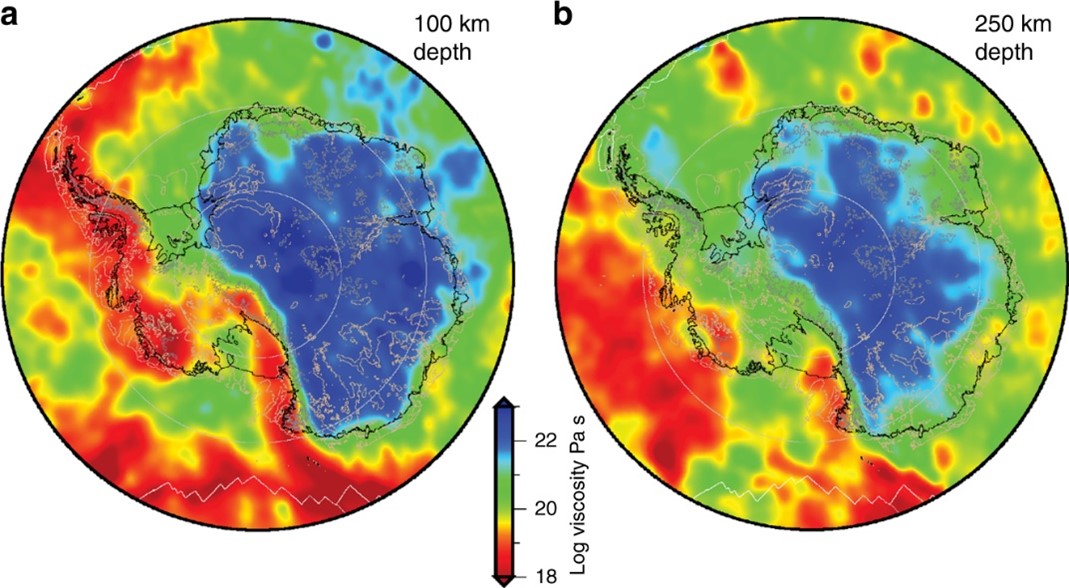
Figure 6: Spatial variations in estimated upper mantle viscosity beneath Antarctica at depths of (a) 100 km and (b) 250 km [Credit: Whitehouse et al. (2019), Nature Communications].
Until recently, it was commonly thought that glacial isostatic adjustment was quite a slow process, taking place at timescales on the order of several millennia (meaning that, if you remove the entire ice sheet, the bedrock would take a few thousands of years to fully recover). However, in the last decade, several studies have highlighted the fact that a mantle way less viscous than initially thought could be observed beneath some areas of Antarctica, and more specifically West Antarctica (such as the Antarctic Peninsula and the Amundsen Sea Embayment — see the red areas on Figure 6 above). Even though it remains very challenging to estimate it with precision, it appears that the viscosity of the mantle lying beneath these regions is particularly (about 2 to 3 orders of magnitude) lower than the average viscosity of the Earth mantle. This would imply very rapid response times of the solid Earth, from only a few years to a few decades, thus way faster than what was previously thought!
What does it mean for the future of Antarctica?
It appears that some of the most extreme low viscosities are observed in the regions under the Thwaites and Pine Island glaciers, where unstable retreat might already be underway! Bedrock rebound in this area might thus be way quicker than we previously thought and could significantly help slowing down ice loss arising from these unstable glaciers. In contrast, quite high viscosities are observed beneath East Antarctica (Figure 6), implying that the stabilising effects of glacial isostatic adjustment would be less efficient in this area.
So now, the main question is: will the uplift be fast enough to stop the ice sheet retreat that we triggered today with current global warming? According to some studies, the low viscosities under the Amundsen Sea Embayment might produce a bedrock uplift large enough and early enough to prevent the complete collapse of the West Antarctic ice sheet, even under strong climate forcing. To properly answer that question, model projections are currently trying to take into account the particular viscosity pattern observed beneath the Antarctic continent, with low viscosities beneath West Antarctica, and reproduce the quick isostatic rebound in this area. But this is cutting-edge research, and work is still in progress. Although the physics of glacial isostatic adjustment is now well understood, the mantle viscosity under the Antarctic ice sheet remains poorly constrained. To be continued…
Edited by Clara Burgard
Mickaël Lalande
I didn’t know that isotactic rebound could be fast enough to stabilize West Antarctica, that’s a great news if this assumption happens! Great article, thank you! 👏