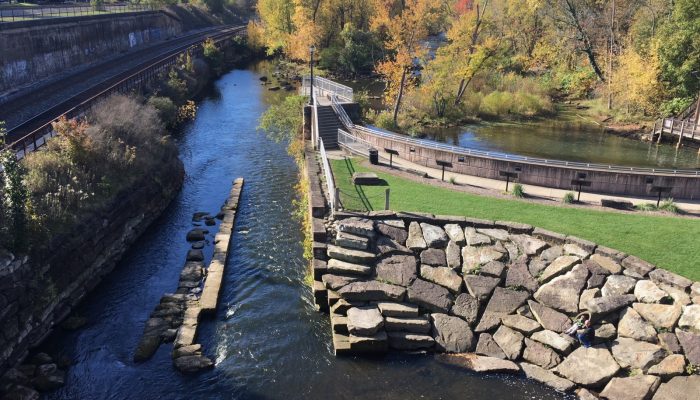
Post by Anne Jefferson, associate professor in the Department of Geology at Kent State University, in the United States.
__________________________________________________
The title of this blog post might seem like a question with an obvious answer, or even a silly question to pose on a blog devoted to groundwater, but if you don’t see the connection between streamflow and underground water, you need to keep reading.
Water in streams during storms does come from the sky, but often it’s not the water droplets falling in a particular rain storm that are going rushing down the stream during that storm. Very little precipitation falls directly on the stream channel, because streams occupy very little of the landscape. So most water in a stream has to travel over or under the ground to get there. Traveling from some random point in a catchment to a stream takes time, and there are usually other water molecules between the freshly fallen raindrop and the channel.
Water molecules that can’t infiltrate into the ground become surface runoff. Surface runoff tends to reach the stream channel fairly quickly, because the water molecules encounter much less flow resistance than water moving through soil or bedrock and because all of the other water molecules between them and the stream are also on the same downhill express lane to the stream. So a water molecule that becomes surface runoff can reach a stream minutes to hours after the raindrop first hit the land surface.
On the other hand, raindrops that infiltrate into the ground take a much more leisurely approach to reaching the stream. Underground, flow resistance is really high, relative to what surface runoff encounters, because the water molecules have to squeeze through tiny and tortuous open spaces between soil particles, roots, and rock. They may even be trapped by capillary forces or stick to mineral or organic surfaces. Plus, underground, there are lots of other water droplets also slowly making their way to the stream. Being a water molecule that goes underground to reach a stream is not so much taking an express lane, as joining the back of a barely moving traffic jam that stretches as far as the eye can see. In the minutes, hours, and days during which a stream runs high following a rainstorm, that poor underground water molecule may only have moved a few meters to a few hundred meters toward the channel. Indeed, its main function in joining the back of the traffic jam was to give a pressure wave nudge to the water molecules closer to the channel, urge them to get a move on, and increase the rate they discharge into the stream.
With those general concepts in mind, let’s turn our attention to humid, forested landscapes. Most rain will infiltrate into the soil, disappear underground, and join the back of the traffic jam. As a consequence, when we look at the water rushing along in a stream channel, during or shortly after a storm, mostly we are not seeing water that fell from the sky in the storm. We’re seeing the ghosts of storms past. Catchment hydrologists call this “old” water. To put some numbers on it, 60-80% of the volume of streamflow during and immediately following a storm is typically old water. Even at the peak of the streamflow, ~50% of the water in the stream is old water.
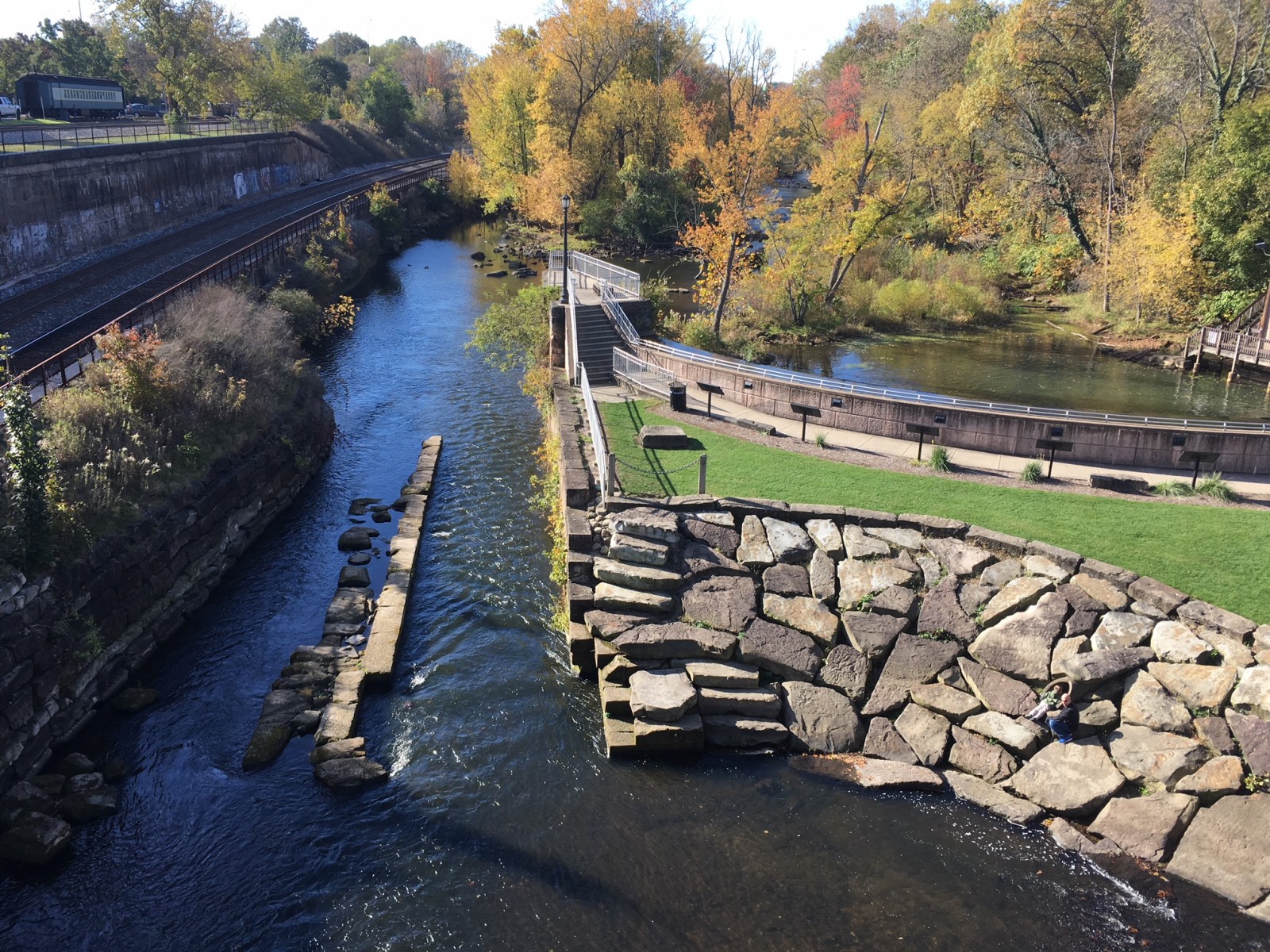
The Cuyahoga River in Kent, Ohio under low flow conditions, October 2017.
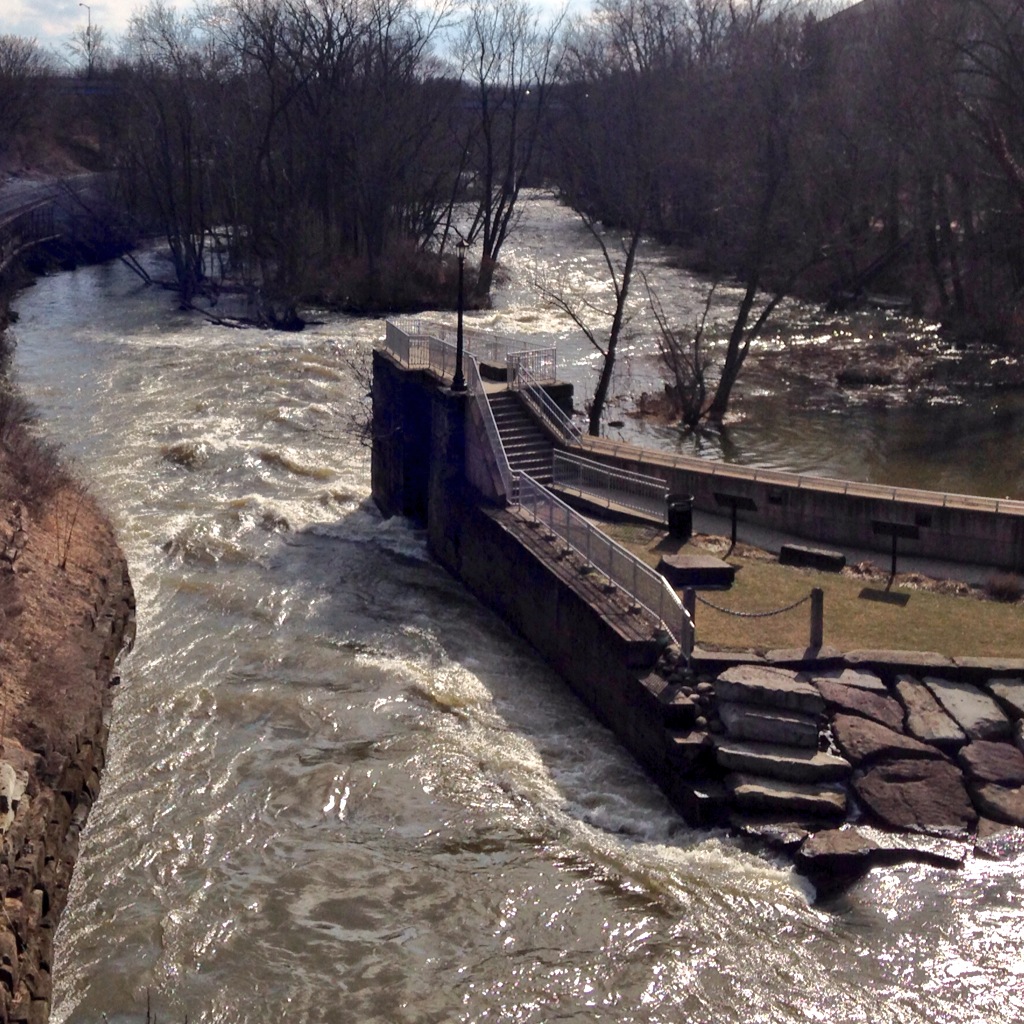
The Cuyahoga River in Kent, Ohio, at high flow, March 2014.
How did hydrologists figure out that the water in streams during storms is old water (at least in forested landscapes)? It would be virtually impossible to go out to a catchment and measure the water flowing across all possible surface runoff, soil water, and groundwater paths.
Wouldn’t it be convenient if there was some way to label the water molecules as new or old and then just count the ratio of new to old water molecules in the stream at a given time? Fortunately for hydrology, variations in the isotopes of hydrogen and oxygen in the water molecules provide just such a tracer. Beginning in the late 1960s, hydrologists realized that they could use the storm-to-storm differences in the isotopic ratios of rainfall to identify water that came from the most recent storm versus water that didn’t. Thus the technique of isotope hydrograph separation was born. The popularity of the method grew rapidly, hydrologists began to try it out in catchments all over the world. This led to more recent work carefully laying out the assumptions and limitations associated with the technique and developing ever more sophisticated methods of analysis. Even more important than the method itself was that hydrologists, confronted with the reality that most of the water in the stream during a storm was old, had to then come up with mechanistic explanations for how that was possible. I don’t think it’s hyperbolic to say that isotope hydrograph separation really helped revolutionize the field of catchment hydrology.
When I teach hydrology, I teach students that in our forested corners of Ohio the water in the stream during storms is old and I explain the mechanisms. But I also think it’s important for them to understand the technique that helped generate those insights, and so I make sure to teach them about isotope hydrograph separation. With the support of an NSF grant, I developed a teaching module that gives students a chance to work with real data and do their own hydrograph separation. My hope is that the exercise will give them a deeper appreciation of the technique, the assumptions and uncertainties it contains, and the insights it is able to provide. This teaching module is available on the SERC website for anyone to use in classes or just for fun. There are data to download, step-by-step directions, and links to related readings. If this post has spurred your interest in streamflow generation or isotope hydrograph separation, I encourage you to check it out. Other good places to learn more about applications of isotopes in hydrology at the SAHRA website or in this book chapter by Kevin McGuire and Jeff McDonnell.
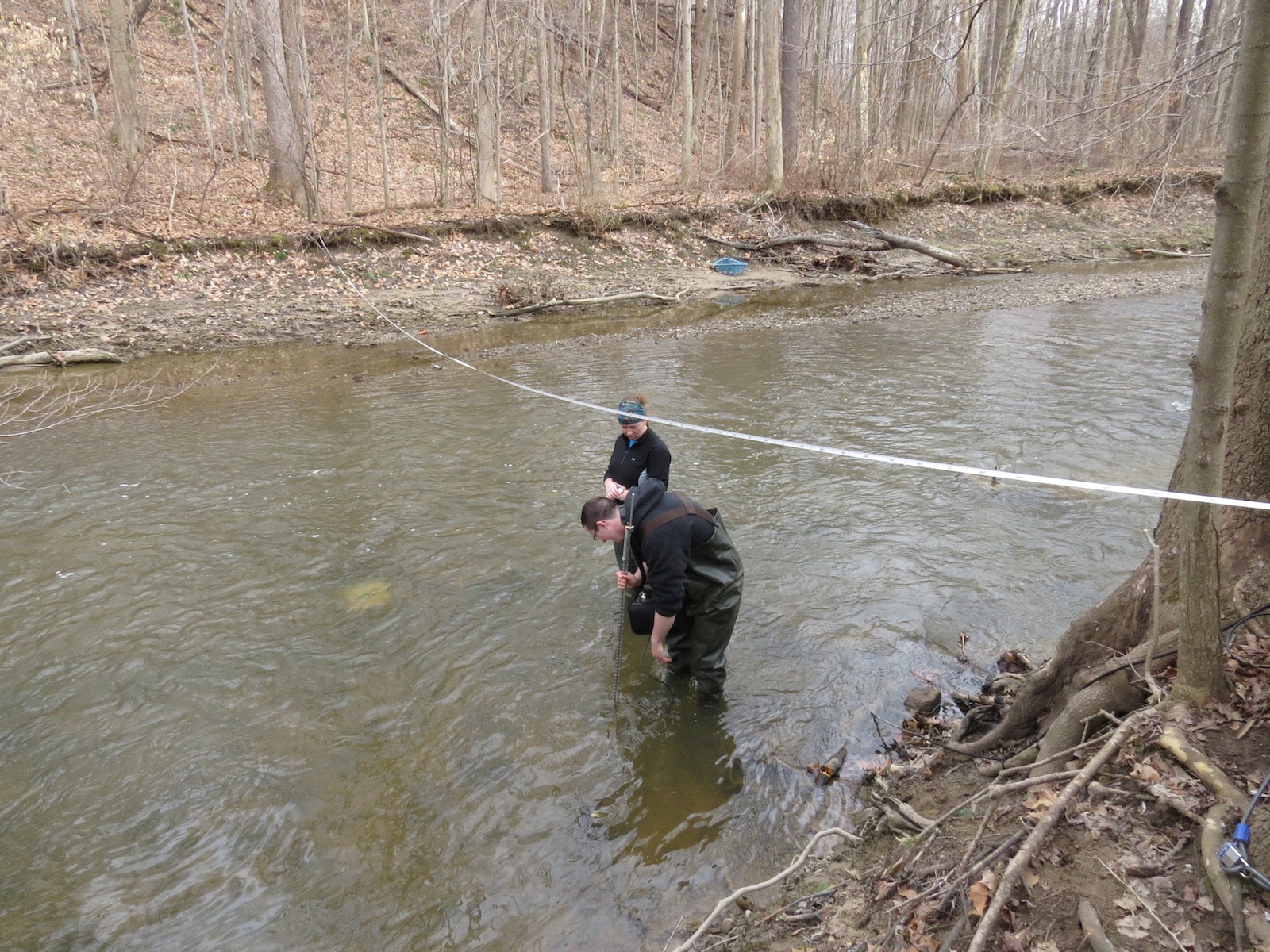
Kent State students measuring discharge in the stream where we conducted the hydrograph separation.
__________________________________________________
Anne Jefferson is an associate professor in the Department of Geography at Kent State University. Anne‘s research focuses on watershed hydrology, groundwater-surface water interactions, and landscape evolution in human-altered and volcanic landscapes. Current projects of her’s focus on green infrastructure, stormwater management, and stream restoration. Keep up to date with Anne by clicking on any of the links below: