Edvard Munch’s series of paintings and sketches ‘The Scream’ are some of the most famous works by a Norwegian artist, instantly recognisable and reproduced the world over. But what was the inspiration behind this striking piece of art? The lurid colours and tremulous lines have long been thought to represent Munch’s unstable state of mind; a moment of terror caught in shocking technicolour. At the ...[Read More]
Extraordinary iridescent clouds inspire Munch’s ‘The Scream’
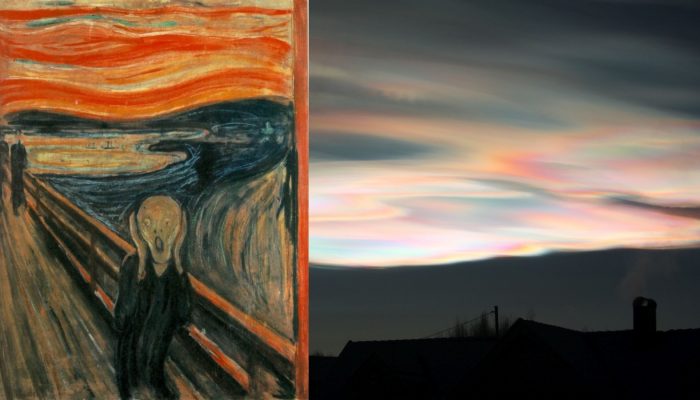