Earlier this month, one of our network bloggers, Matt Herod, put out a call for posts on momentous discoveries in geology as part of a well-known geoscience blog carnival, The Accretionary Wedge. With so many geoscience disciplines to choose from, and an immense wealth of exciting discoveries across the Earth sciences, choosing just one momentous discovery was no easy task. Much of my background is in oceanography though, and while momentous moments mark much of the field’s history, the CTD stands out – to me at least – as one of oceanography’s most sterling inventions.
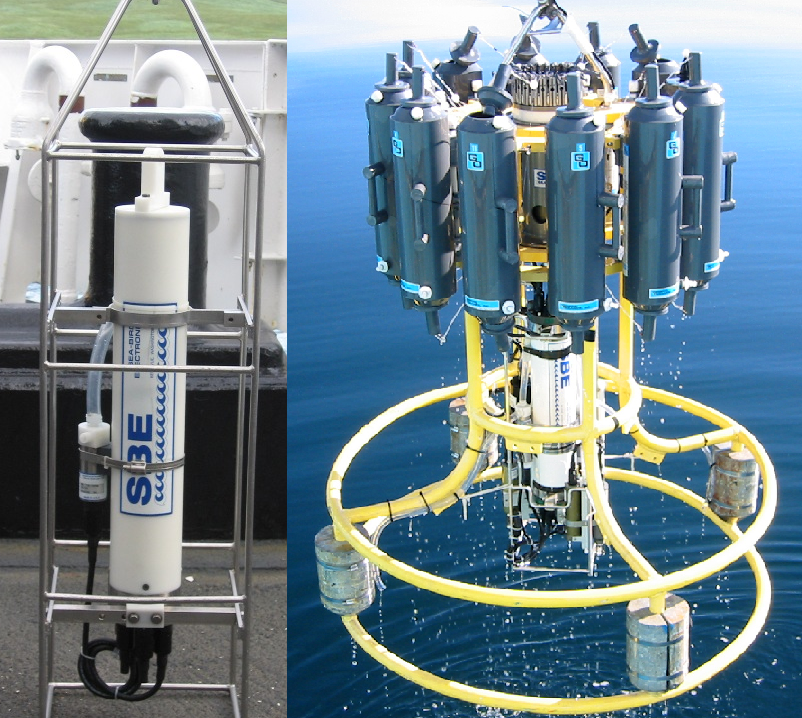
This is a CTD (left). Often deployed off research ships with a large bundle of sampling bottles known as a rosette (right), CTDs record the conductivity (and hence, salinity), temperature and depth of water in the ocean, allowing physical oceanographers to get a good look at it’s structure and work out which water masses are moving where. (Both the CTD and rosette images are credited to NOAA)
Conductivity, Temperature and Depth recorders (CTDs) provide the data that forms the foundation of physical oceanography. Dissolved salts carry a charge and this charge can be detected using a conductivity meter, the “C” in our CTD. From this, oceanographers can determine the salinity of the water. Why do we want to know this? Salinity can be used to trace different water masses as they mix in the ocean. For example, the heat of the Mediterranean region causes large quantities of water to be evaporated, leaving a very salty sea behind. Consequently, when water leaves the Mediterranean Sea and enters the Atlantic, it has a distinctly salty signature. On entering the Atlantic, it sinks because it is more salty – and hence more dense – than the surrounding water. This body of water sits midway between the ocean surface and seafloor, so is known as Mediterranean Intermediate Water (MIW).
Temperature too, has a role to play in water density, where warm tropical waters are less dense than the cold waters at the poles. Cold water in the North Atlantic sinks to form a mass of water known as North Atlantic Deep Water (NADW), which descends from the Arctic to the seafloor and spreads south along the floor of the Atlantic Ocean. The same happens in the Southern Ocean, where cold Antarctic water sinks and spreads north, forming Antarctic Bottom Water (AABW). Temperature can also be used as an indicator for where water masses originated, but identifying them requires a little tweaking of the data. This is because water heats up as it is compressed, so as a water mass sinks, the increasing pressure exerted by the water above it causes it to warm up and obscures its original temperature signature. Fortunately, the relationship between temperature and pressure is a well known one, and scientists can correct for this heating if they know the depth of the water mass.
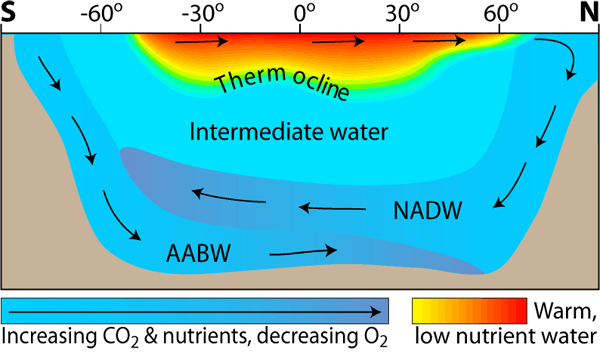
Simplified water circulation in the Atlantic Ocean. Temperature and salinity dictate the density of a water mass and thus, its position in the water column, with warmer, fresher water at the ocean surface and colder, more saline water at depth. (Credit: SEOS)
Depth can be determined using pressure sensors in the CTD, and it’s with these that we can work out where each of the water masses (with their unique salinity signatures) are located, and where their boundaries with other water masses are too. CTDs are lowered through the water column to determine how temperature and salinity vary with depth, but to find out how these parameters also vary with space, oceanographers have to drop CDTs all over the ocean. Well, sample it at least. By regularly deploying CDTs at different locations, oceanographers can work out where water masses are in space, as well as depth.
Circulation patterns like the one above happen in oceans over the world and together these great moving masses of water make up a worldwide ocean circulation system known as the thermohaline conveyor belt, which influences rainfall patterns, climate, nutrient mixing, fish stocks and so much more. The uncovering the thermohaline conveyor belt in itself is a momentous discovery, but how do we know about it? The CTD. Amazing.
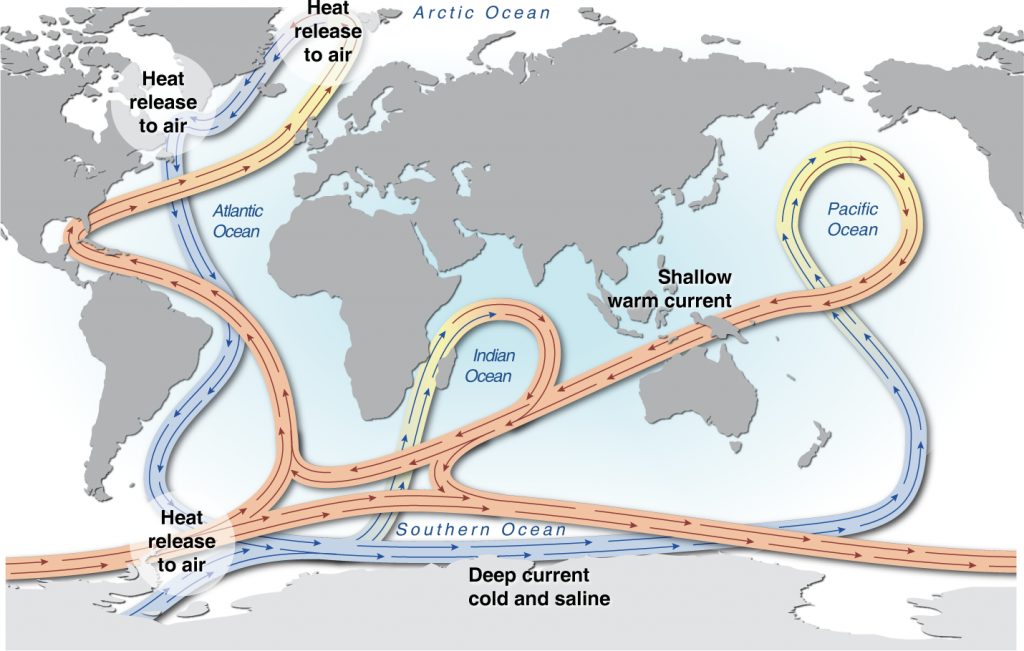
The global ocean conveyor belt. Blue indicates the movement of cold deep water masses and red indicates the warm surface currents that make up the oceans’ thermohaline circulation system. (Credit: UNEP)
By Sara Mynott, EGU Communications Officer
Md. Sohel Parvez
Thank you Sara Mynott, for writing so nicely the uses and role of CTD in the study of Oceanography. I also astonished with the magical outputs of this small device from a single operation.