Today in GeoTalk, we’re talking to Suzanne Hangx, who explains the great potential of carbon capture and storage and the challenges emerging technologies, like CCS, face.
First, could you introduce yourself and let us know what drew you to geomechanics?
Let’s start with the introduction: I’m Suzanne Hangx and I currently work as a researcher on geomechanics for subsurface storage containment technologies at Shell Global Solutions in the Netherlands. Actually, I hadn’t planned, or even thought of, becoming a geomechanist at first. In high school, I was mainly interested in chemistry and I was thinking of studying Chemical Technology. It wasn’t until I read a book about volcanoes for Geography class that I thought: “maybe I want to become a volcanologist!” To me it nicely combined chemistry, travelling and Earth processes, which I also found interesting. So I enrolled for my geology studies at Utrecht University. As it turned out, volcanoes were not my cup of tea, but I did meet a great professor that introduced me to geomechanics. All of a sudden I had found it: breaking rocks (for scientific purposes of course… and also a bit for fun) was my ‘thing’.
After finishing my Master’s in 2004, I continued working with Professor Chris Spiers at the High Pressure and Temperature Laboratory at Utrecht University. I started a PhD project on CO2 storage and the effect of chemical CO2-rock interactions on the mechanical properties of rocks. To me that was a nice combination of putting science in a socially-relevant context, as CO2 storage is considered to be a potential solution to reduce greenhouse gas emissions. When I finished my PhD in 2009, I got the opportunity to continue this work at Shell Global Solutions.
Last year, you received a Division Outstanding Young Scientists Award for your work on Carbon Capture and Storage (CCS). Could you tell us about your research in this area?
One way to get rid of large quantities of greenhouse gasses, like CO2, is to inject them into the subsurface, reducing their effect on climate change. Suitable locations are depleted oil or gas reservoirs, or aquifers, at several kilometres depth. However, it is key to ensure that after injection the CO2 also stays there – not just today or tomorrow, but for thousands of years. Once a potential injection site is identified, it is important to see if the reservoir (the ‘container’) and the seal keeping the CO2 in place (the ‘lid’), are up for the job, so to speak. I investigate if the injected CO2 does anything to the rocks to alter their mechanical behaviour, i.e. how they break, under which force they break and if they get weaker. Once I have this data, I will give it to the people that make the long term (thousands of years) numerical modelling predictions of the behaviour of the reservoir-seal system. This way, we all work together to determine whether or not a potential site is suitable for geological storage of CO2.
How does carbon dioxide affect the chemical and mechanical properties of rocks?
When you inject CO2 into a depleted oil or gas reservoir, part of it will start to dissolve into the water that is present in that reservoir, while the rest will stay in a dense liquid or supercritical phase. When CO2 dissolves in water, the water will become acidic. This acidic fluid can chemically interact with the surrounding rocks – like in fizzy drinks, where CO2 is injected to make them fizz, but they also become acidic and corrode your teeth if you drink too much of them. As a result, certain minerals may dissolve and new ones may be formed, also the way cracks propagate through the rock may be affected, changing their strength and the way they break. If a rock gets sufficiently weakened by the chemical interaction with CO2 it may compact or break. As a result, a fracture may be created through the seal and CO2 could leak out of the reservoir. This is something we absolutely do not want, and therefore, for every new, potential site we look at how CO2 can affect the rocks of that specific location in great detail.
At the same time, chemical interactions may occur on different timescales. Processes that happen in days, weeks or months can still be dealt with in a laboratory setting. However, to be able to predict what will happen on the timescale of thousands of years, we are currently trying to learn as much as we can from naturally occurring CO2 fields, such as those in Utah (USA), Australia and Europe. These fields can contain over 90% pure CO2 and have mostly done so for millions of years. At Shell, we have recently set up a research consortium with international universities and research institutes to investigate the chemical, mechanical and transport properties of a natural CO2 field in Green River, Utah. The results from this program can help us understand better how anthropogenic CO2 injection into the subsurface will evolve over time.
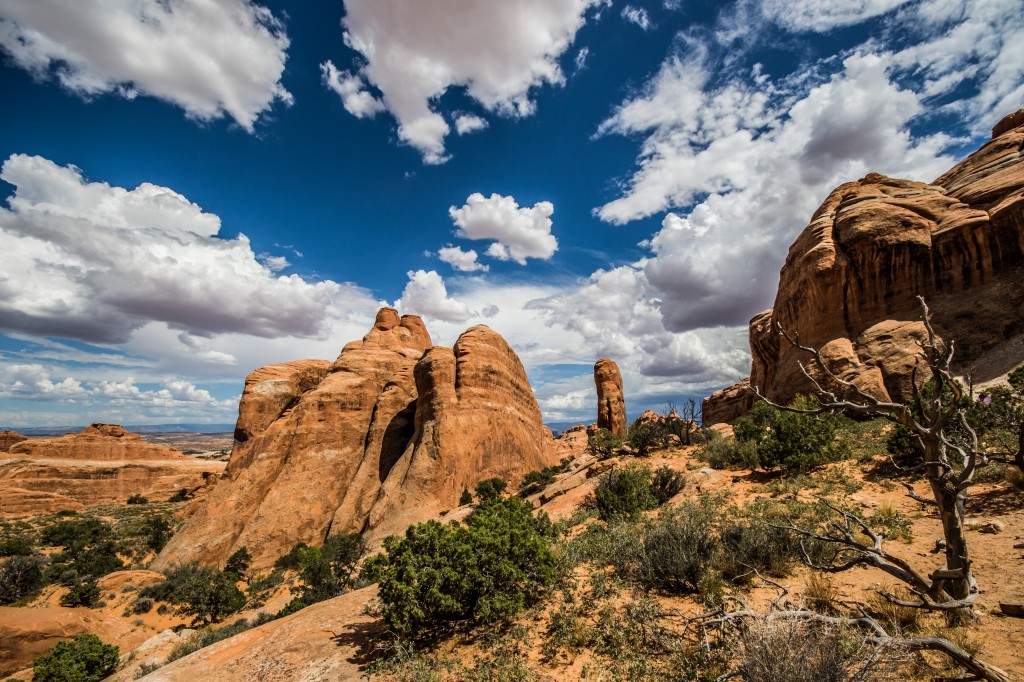
In some areas in Utah (USA), subsurface rock formations of the Entrada Sandstone have held natural CO2 accumulations for millions of years. (Credit: Suzanne Hangx)
Under what conditions can caprock integrity be compromised, and how can we avoid this?
Loss of caprock integrity, i.e. a leaking ‘lid’, can occur if you try to inject too much CO2 into a reservoir. As a result, the pressure in the reservoir may get too high and will try to escape, usually along the path of least resistance. To avoid overpressuring of the injection site, strict regulations are set to injection volumes and pressure. It is not allowed to inject CO2 at a higher pressure than the original oil or gas pressure in the reservoir, before production started. We strive to stay well below this value to make sure we do not induce failure of the seal.
Integrity can also be compromised by chemical weakening of the seal by the injected CO2. Here, laboratory experiments and studying the seals of natural CO2 field helps to understand what processes we need to look at and pay attention to, before we can say that a seal is good enough.
What technical challenges face CCS and how do you think we can overcome them?
The CCS chain consists of CO2 capture at source, followed by transport to the site, and then injection into the reservoir for storage. For storage, we already understand the larger part of the processes that are of importance. Key challenge is still to investigate every new site in great detail, which is very time consuming and could take several years. In addition, it is also about getting the general public to accept this technology as a way to battle climate change.
Finally, what do you hope to work on next?
I’m mainly driven by my curiosity about the physical and chemical processes that control rock material behaviour in the subsurface, along with the direct relevance of this field to socially relevant issues. With energy and water demand rapidly increasing globally, while availability continues to diminish, densely populated areas are becoming increasingly targeted for exploitation. We already notice that in some areas oil, gas and ground water pumping leads to surface subsidence and induced seismicity. In addition, hydraulic fracturing (‘fracking’) of subsurface reservoirs for shale gas and geothermal exploitation meets with strong public opposition, due to the risk of induced ground motion, seismicity, and health and safety hazards, some of which are miscommunicated. I would like to contribute to such geo-energy problems, by investigating and quantifying these risks and coming up with socially acceptable solutions or technologies.
If you’d like to suggest a scientist for an interview, please contact Sara Mynott.