We are pleased to propose you a new Paper of the Month written by Dr. Derek Keir on volcano seismology. Derek’s PhD thesis was on the “Seismicity of the Ethiopian rift” and conducted at Royal Holloway University of London under the supervision of Prof. Cindy Ebinger and Prof. Graham Stuart of the University of Leeds. Towards the end his PhD studies, the Dabbahu rifting episode started (September 2 ...[Read More]
Paper of the Month – Bubbles and seismic waves
Our paper of the month is “Bubbles attenuate elastic waves at seismic frequencies: First experimental evidence” (N. Tisato et al., 2015) commented by Luca De Siena. Luca De Siena is Lecturer in Geophysics at the School of Geoscience, University of Aberdeen (UK). He received his PhD from the University of Bologna (Italy) with a scholarship from the INGV-Osservatorio Vesuviano for his work on seism ...[Read More]
Paper of the month — Signal apparition for wavefield separation
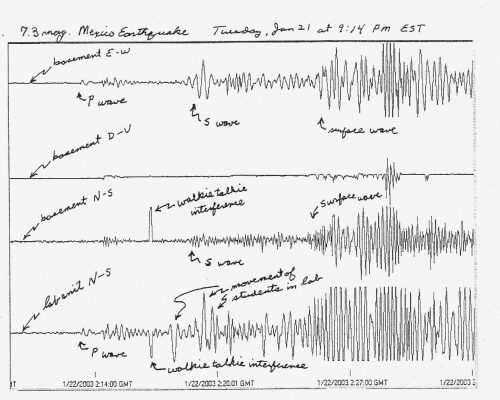
Our paper of the month is “Signal apparition for simultaneous source wavefield separation” (J. Robertsson et al., 2016) commented by Andreas Fichtner. Andreas Fichtner is Assistant Professor for Computational Seismology at the Swiss Federal Institute of Technology (ETH) in Zurich. He received his PhD from the University of Munich for his work on Full Seismic Waveform Inversion for Str ...[Read More]
Paper of the Month — Seismic anisotropy
“SEISMIC ANISOTROPY AND MANTLE DEFORMATION: WHAT HAVE WE LEARNED FROM SHEAR WAVE SPLITTING?” (M. K. SAVAGE, 1999) commented by Dr. Jessica Johnson Jessica Johnson from the University of East Anglia (UK) is our guest author of the PoM blog series of this month! She has chosen to comment on the paper “Seismic Anisotropy and mantle deformation: what have we learned from shear wave ...[Read More]