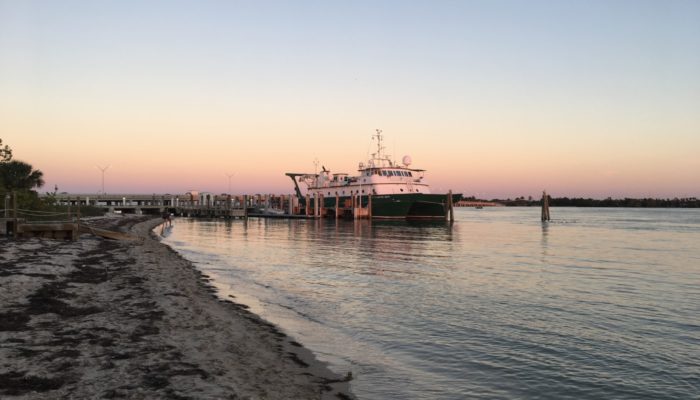
In the ocean, mesoscale eddies are everywhere. In fact, about 90% of the ocean’s kinetic energy is contained within these ubiquitous, ~100 km swirling vortices. We know how these eddies form, through a process known as baroclinic instability, where density surfaces are tilted too steeply and become unstable. What remains unclear however, is what happens to this energy when eddies die. This knowledge gap is a key uncertainty in ocean/climate models that parameterise ocean eddies, like the state-of-the-art CMIP6 models used in the latest IPCC report.
What we do know however, is where these eddies typically go to die: ocean western boundaries. Using satellite-based altimetry we can observe westward propagating mesoscale eddies as they reach ocean western boundaries, like the east coast of the United States, and disappear. What happens to this eddy energy at western boundaries then becomes a question of the direction of the energy cascade. An eddy may become entrained within the larger scale flow features of the region in what is known as an inverse or reverse energy cascade (from mesoscale to large scale). Alternatively, an eddy may interact with the topography along the ocean boundary, forming smaller scale features that eventually dissipate as turbulence. This is what we call a direct or forward energy cascade.
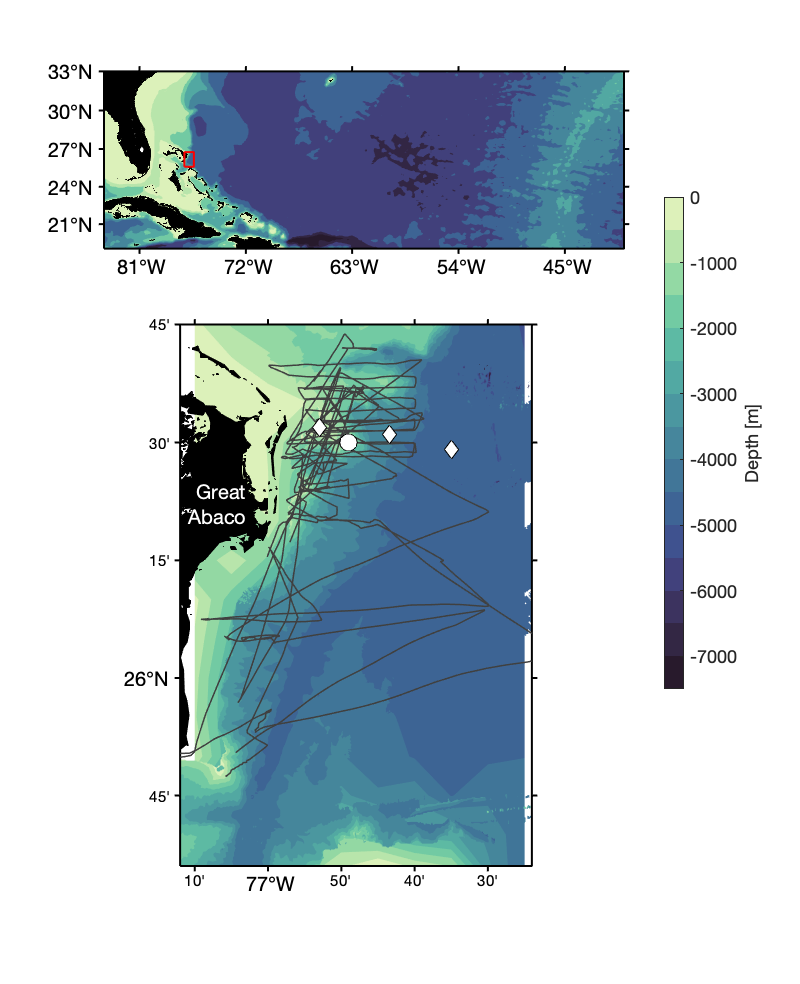
The MeRMEED study region offshore of Great Abaco. The grey lines show location of our survey and the white markers show the location of existing moorings. (Fig. 2; credit: Gwyn Evans)
During my post-doc at the University of Southampton, I was part of the MeRMEED (MEchanisms Responsible for Mesoscale Eddy Energy Dissipation) project led by Eleanor Frajka-Williams. We set out to observe and quantify the strength of the direct energy cascade in mesoscale eddies at an ocean western boundary by measuring the turbulence generated as eddy flow interacts with topography. Our chosen study region was just offshore of Great Abaco, Bahamas, where we already have observations of possible eddy-topography interactions from mooring-based measurements and satellite altimetry (Fig. 2). Over the course of two years, the plan was to use a relatively small vessel to perform a short but intensive survey of the flow and turbulence along the steep and rough slope offshore of Great Abaco during several different eddies.
To understand how eddy flow-topography interactions can lead to an increase in turbulence and an eventual dissipation of eddy energy, we required detailed measurements of two key variables: the sub-surface velocity and the turbulent dissipation. For sub-surface velocity measurements, we used an Acoustic Doppler Current Profiler or an ADCP. Our chosen vessel, the R/V F.G. Walton Smith of the University of Miami (Fig. 1), came equipped with an ADCP mounted to the hull of the vessel, allowing us to perform a comprehensive survey of the eddy flow offshore of Great Abaco. To complement these measurements, we used a Vertical Microstructure Profiler or VMP to estimate turbulent dissipation (Fig. 3). The VMP is specialist piece of equipment that measures centimetre to millimetre scale changes in vertical gradients of velocity and temperature. By examining the spectra of these small-scale changes, we can then make estimates of the turbulent dissipation to tell us how much of the eddy flow’s kinetic energy is dissipated along the western boundary offshore of Great Abaco.
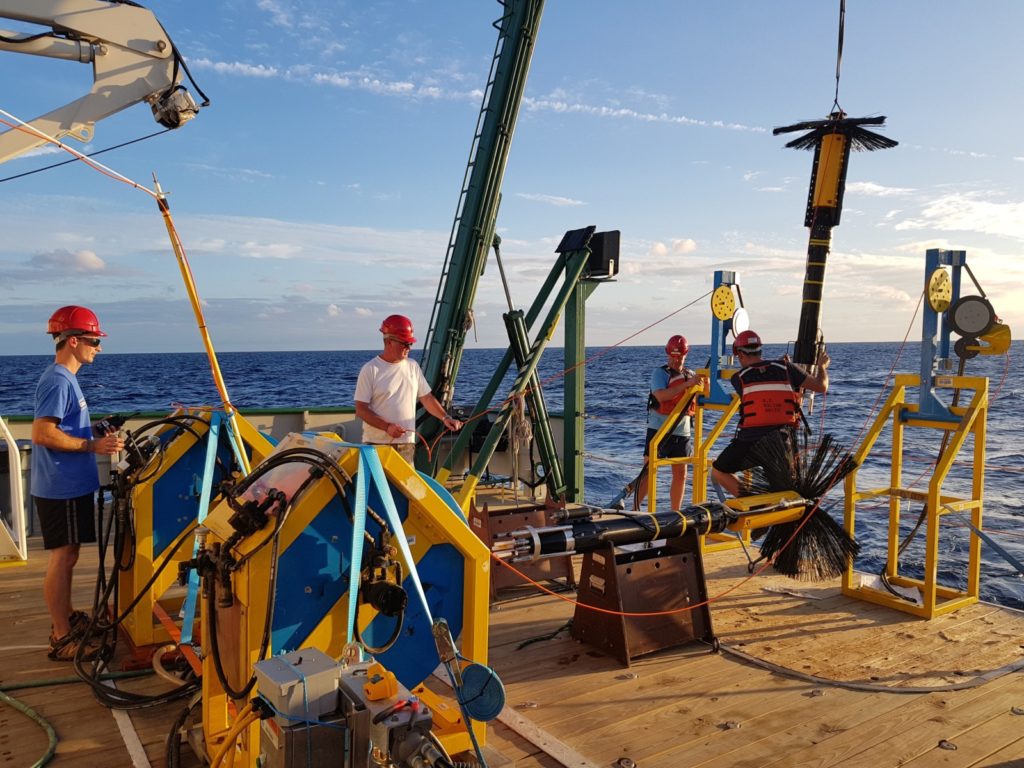
The VMP in action during a recover on the aft deck of the R/V F.G. Walton Smith handled expertly by colleagues from the National Marine Facilities group of the National Oceanography Centre. (Fig. 3; credit: Eleanor Frajka-Williams)
Our measurements across three different mesoscale eddies revealed a whole host of eddy flow-topography interactions that led to increased turbulence and dissipation of the eddy flow’s kinetic energy. For example, we observed several regions of strong vertical and horizontal gradients in velocity linked to interactions between the eddy flow and topography where we also observed increased turbulence. Turbulence was also stronger where the eddy flow is forced over bumps in the topography including the generation of internal gravity waves, which are linked to the presence of a hydraulic jump. Our work has therefore shown us that the interaction between eddies and the steep and rough slope at an ocean western boundary can lead to turbulent energy dissipation within the eddy flow. However, does the energy dissipation observed during our surveys explain the decay of eddy energy observed at western boundaries via satellite altimetry?
To answer this question, we again used satellite altimetry to track every eddy that entered our study region and “died”. We estimated the energy of these eddies and monitored the decay of their energy over time within our study region. Comparing this satellite-based estimate of eddy decay rate to the turbulent dissipation measured during our surveys, we find that these separate estimates agree very closely. This suggests that offshore of Great Abaco, eddy energy is lost through a forward cascade of energy, as the mesoscale eddy flow interacts with the steep and rough slope, forming smaller scale features that eventually lead to the generation of turbulence and an increase in the dissipation rate.
The MeRMEED project has shown us that the death of mesoscale eddies at ocean western boundaries can in some cases be explained through a forward cascade of energy, where eddy-topography interactions lead to smaller scale flow features that eventually dissipate as turbulence. The question remains, however: are our results relevant for other ocean western boundaries beyond this particular region offshore of Great Abaco? And further, how should these findings inform eddy parameterisations in the next generation of ocean/climate models? These remain open questions that will no doubt guide the future research within this field.