The Weddell Seal pops his head up through the hole in the floor of the shipping container… for the fourth time today. The shipping container is one of several making up our field camp on sea ice, 40 km from Scott Base – situated on Ross Island, in the south-western Ross Sea. Today I talk about the sub-ice platelet layer, which provides the base for a rich marine environment. Generating super ...[Read More]
Image of the Week – Receiving messages from the deep
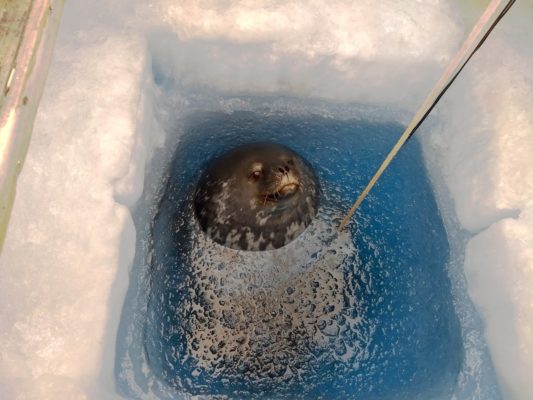