New Year’s Eve is just around the corner and the last “image of the week” of 2016 will get you in the mood for a party. If your celebration needs a soundtrack with a suitably geeky touch then look no further. Here is the music for climate enthusiasts: The sound of the past 60,000 years of climate. Scientist Aslak Grinsted (Centre for Ice and Climate, University of Copenhagen, Denmark) has transfor ...[Read More]
Image of the Week – The Sound of an Ice Age
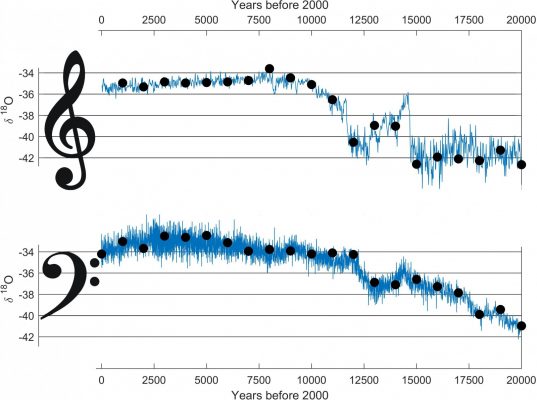