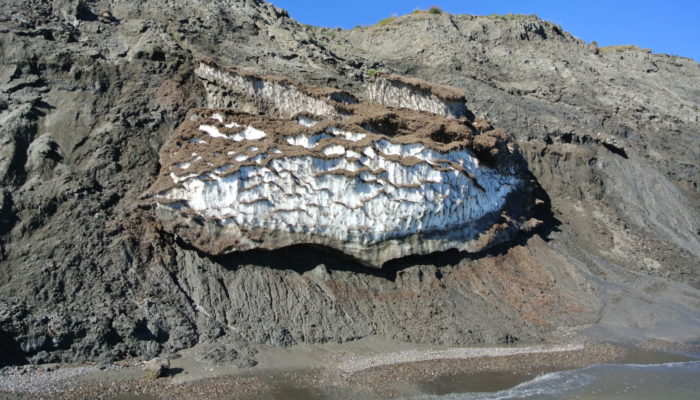
Nowadays, there are plenty of media reports about the impacts of climate change around the world. Glaciers are disappearing, gigantic craters form in Siberia as the previously frozen ground thaws, the sea is threatening to swallow entire islands, floods cause large damages to people and economy, heat waves periodically destroy crops and can reach dangerous levels for people’s health. And this is only the “tip of the iceberg” in terms of climate change-related catastrophic events. Indeed, there is a broad spectrum of phenomena occurring at different spatiotemporal scales, from the sudden and localized episodes of extreme precipitation events to the global, decade-long variations in sea level rise. It is also common for the media to explain the link among these different phenomena as the effect of global warming. Nevertheless, this notion is not accurate, and scientists explain these effects, together with global warming itself, as consequences of global heating.
Actually, the concept of global heating is rather simple: before the Industrial Revolution, the energy reaching the Earth from the Sun was balanced by the emitted infrared radiation into Space, thus the climate was steady in the long-term. But after changing the atmosphere composition by releasing large amounts of greenhouse gases, the emitted infrared radiation decreased. As a consequence, an energy imbalance developed, since the incoming Solar radiation has been approximately the same in the last century (within the well-known cycles of solar activity). Thus, the Earth starts to accumulate energy in form of heat, since more energy is now being absorbed by the planet than it is released into Space, leading to a situation of global heating.
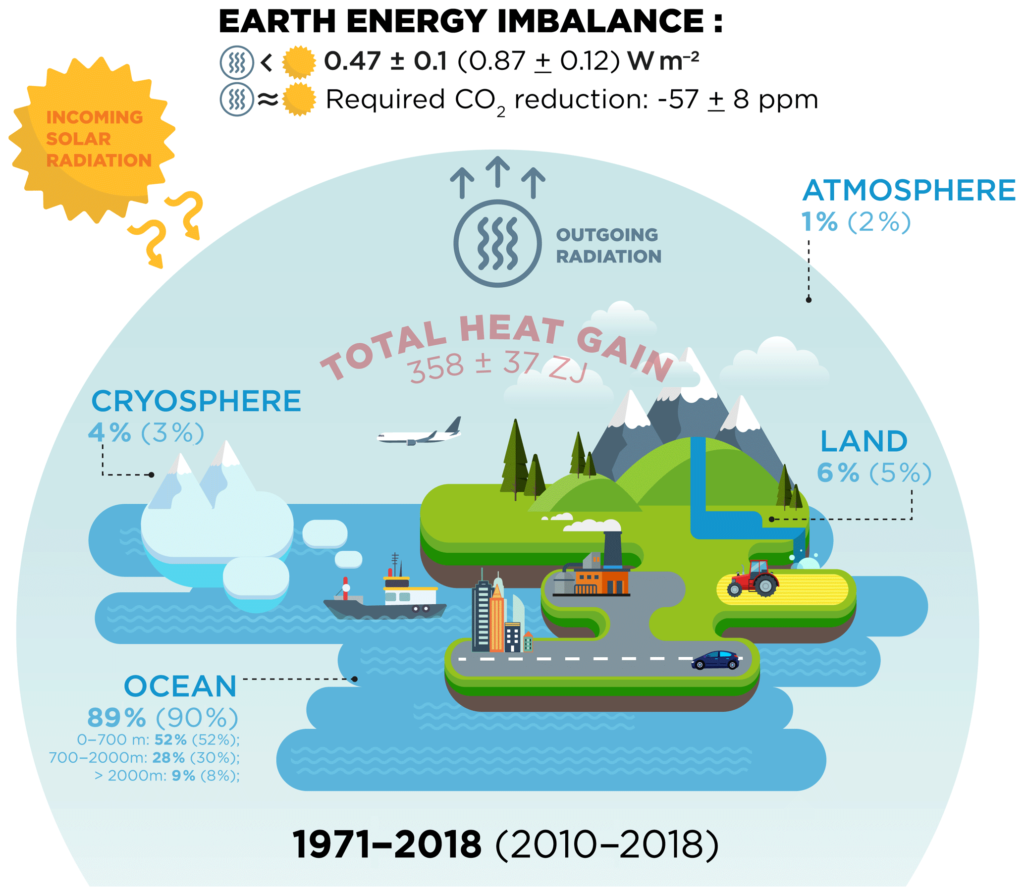
Figure 2: Observed proportion of heat storage in the ocean, the continental subsurface, the atmosphere, and the cryosphere. Bold text indicates estimates for the period 1971-2018, normal text indicates estimates for the period 2010-2018. Source: Ref. 1.
Sure, heating is a fancy word, but what does it means for the planet? How is this related to sea level rise, heat waves, or floods? Let’s start from the beginning. The planetary heating just means that the Earth is going to store heat. This heat storage happens by changing the temperature of the ocean, the atmosphere, and the continental subsurface, and by melting ice. The heat distribution among the oceans, the atmosphere, the continental subsurface, and the cryosphere have been assessed recently by an international collaboration of scientists [1] using all measurements available: meteorological observatories, buoys, satellite data, subsurface temperature profiles, shipping records, and more. The vast majority of the extra heat in the Earth goes into the ocean (89% of all stored heat, see Ref. 1), increasing its temperature and expanding its volume. This ocean expansion is one of the causes of sea level rise. The other main contributor to sea level rise is ice melting of land glaciers and ice sheets in Greenland and West Antarctica, adding water to the ocean. Melting glaciers and ice sheets, together with melting sea ice, constitutes the third largest heat reservoir of the Earth (4%), while the continental subsurface stores the second largest amount of heat (6%). Ground heat is divided mainly into warming the global subsurface and thawing permafrost soils in the Arctic and the Tibetan Plateau. This is the main reason for the craters in the Arctic region: once the previously frozen subsurface thaws, it allows soil bacteria to process now accessible organic matter, releasing gases that remain trapped underground. When the accumulation of gases is large enough, an explosive release of gases can occur forming the crater. The remaining 1% of heat is stored in the atmosphere, warming the air. Note that warmer air is able to carry more water vapor from the ocean into the land, which can make floods and extreme precipitation events more frequent and dangerous in some regions, such as India. Furthermore, the warming of the air temperatures induces more frequent heat waves in already warm and dry areas of the world, such as around the Mediterranean Sea.
Therefore, global heating can be seen as a metric of climate change. That is, since dangerous climate phenomena for society are associated to heat storage, the future amount of accumulated heat will inform us about the evolution of these dangerous phenomena. But how much more heat will the Earth store towards the end of the century? In order to answer these questions, we need to use climate models. Climate models are just collections of knowledge and hypotheses. Models depict the natural laws we know, such as gravity, how the air moves, or heat transfer from hot objects to cold ones, among others. We put all these laws together and we ask the model “what if” questions. What if we change the atmospheric concentration of greenhouse gases? What if we cut the Amazon Forest? What if a significant part of the Antarctic ice sheet suddenly collapses? Climate models are not designed to predict the weather in a specific day ten years in the future, but to provide a general probabilistic picture of climate. However, we are not able to include all the natural laws into a climate model, as the required computing power would be outrageously large, and therefore we can only include the most important processes for the evolution of climate. Moreover, the mathematical methods to tell a computer what the natural laws are have some unavoidable limitations, thus we need to approximate certain processes instead of mathematically solving them in the models.
But before we can ask a model any question, we need to know, first of all, if the model is suitable to answer our questions. To this end, we evaluate the models against observations of climate variables such as temperature evolution, precipitation patterns, or wind regimes. In our study [2], we have assessed the ability of the models used in the last Assessment Report of the Intergovernmental Panel on Climate Change (IPCC) in 2014 to reproduce the recent observations of heat storage in the ocean, continental subsurface, cryosphere, and atmosphere.
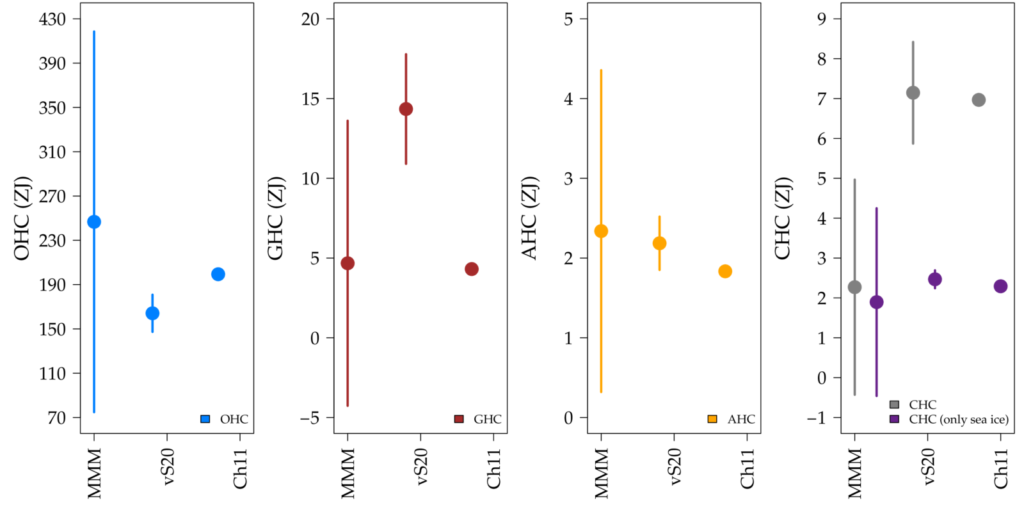
Figure 3: Heat storage within the ocean (OHC, blue), the continental subsurface (GHC, brown), the atmosphere (AHC, yellow), and the cryosphere (CHC, gray) from models (MMM label) and observations (vS20 [1] and Ch11 [3] labels). Purple dots indicate the heat storage by sea ice. Source: Ref 2.
The models simulate an excessively large amount of heat in the ocean in comparison with observations, and excessively small amounts of heat in the continental subsurface and the cryosphere. Models and observations present similar values of heat storage in the atmosphere. Do these results mean the models are wrong? Not necessarily, models are incomplete, as they do not include all known physical laws. If the very same models analyzed here included deeper continental subsurfaces in their land surface components and more physical processes, they would do a much better job in reproducing observations of continental heat storage. Similarly, if these models included some representation of terrestrial ice masses, such as glaciers and ice sheets, the simulated cryosphere heat content would be much more similar to the observed heat storage. In fact, models are able to reproduce the observed heat storage due to sea ice melting, which is much better represented in the models than the land ice masses. And what about the large amount of ocean heat content simulated by the models? This is a rather complex subject; the cause may be an excessively high response of the models to the prescribed emissions of greenhouse gases. In fact, the magnitude of the climate response to greenhouse gases is still a debatable subject, as there are large uncertainties accounting for the estimated responses of both measurements and model simulations [4-5].
It is important to note that the reason for the incomplete depiction of the Earth system in these climate models is that building more complete models have a larger computational cost. Modelling centers balance the degree of complexity of their models with the research objectives they pursue. For example, some of the analyzed models in Ref. 2 were used to simulate climate conditions during the last 1150 years, which requires a high amount of computational resources. Therefore, scientists balance the level of detail represented in models in order to invest those precious resources in knowing better the long-term variations of climate in the past. Although scientists need climate models that can simulate distant epochs in the past as well as long-term periods in the future, including a better representation of certain physical processes in these models is required to improve their simulated heat storage and heat-dependent processes. The current increase in computational resources and new mathematical techniques to represent physical processes may allow to enhance the level of detail in the next generations of climate models, while maintaining their ability to produce global climate simulations at millennial time scales.
This post has been reviewed by the editorial board.
References [1] von Schuckmann, K., Cheng, L., Palmer, M. D., Hansen, J., Tassone, C., Aich, V., Adusumilli, S., Beltrami, H., Boyer, T., Cuesta-Valero, F. J., Desbruyères, D., Domingues, C., García-García, A., Gentine, P., Gilson, J., Gorfer, M., Haimberger, L., Ishii, M., Johnson, G. C., Killick, R., King, B. A., Kirchengast, G., Kolodziejczyk, N., Lyman, J., Marzeion, B., Mayer, M., Monier, M., Monselesan, D. P., Purkey, S., Roemmich, D., Schweiger, A., Seneviratne, S. I., Shepherd, A., Slater, D. A., Steiner, A. K., Straneo, F., Timmermans, M.-L., and Wijffels, S. E. (2020). Heat stored in the Earth system: where does the energy go?, Earth Syst. Sci. Data, 12, 2013-2041, https://doi.org/10.5194/essd-12-2013-2020. [2] Cuesta-Valero, F. J., García-García, A., Beltrami, H., and Finnis, J. (2021). First assessment of the earth heat inventory within CMIP5 historical simulations. Earth Syst. Dynam., 12, 581-600, https://doi.org/10.5194/esd-12-581-2021. [3] Church, J. A., White, N. J., Konikow, L. F., Domingues, C. M., Cogley, J. G., Rignot, E., Gregory, J. M., van den Broeke, M. R., Monaghan, A. J., and Velicogna, I. (2011). Revisiting the Earth’s sea-level and energy budgets from 1961 to 2008. Geophys. Res. Lett., 38, L18601. https://doi.org/10.1029/2011GL048794. [4] Knutti, R., Rugenstein, M. & Hegerl, G. (2017) Beyond equilibrium climate sensitivity. Nature Geosci 10, 727-736. https://doi.org/10.1038/ngeo3017. [5] Meehl, G. A., Senior, C. A., Eyring, V., Flato, G., Lamarque, J.-F., Stouffer, R. J., Taylor, K. E. and Schlund, M. (2020) Context for interpreting equilibrium climate sensitivity and transient climate response from the CMIP6 Earth system models, Science Advances, 6(26), eaba1981. https://doi.org/10.1126/sciadv.aba1981.