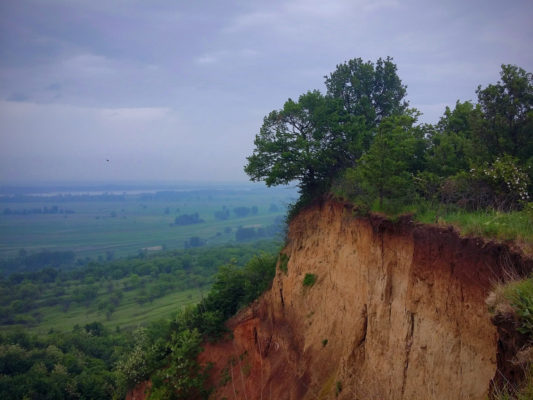
Loess is a mineral, aeolian deposit with a range of definitions in literature, which class it as either a sediment, soil, or rock. Some classic texts suggest that “loess is not just the accumulation of dust” [1], and it must include additional processes such as loessification, calcification, pedogenesis, and in-situ weathering. The definition adopted depends on the scientific background and the question being posed, but my preference is to simply define loess as: an aeolian, mineral (i.e. non organic), silt-size dust deposit associated with a variety of additional, often a site specific processes.
Loess has particular value from the perspective of reconstructing past climates. Typically, oxygen isotopes from ice- and marine- cores are used to investigate long-term Quaternary changes, but they lack information about terrestrial processes and environments. Lacustrine cores and speleothems hold fantastic long-term records (in some cases thousands of years [2,3]), but they are often limited to very specific depositional conditions and can be discontinuous (especially >40° north and south). Loess on the other hand covers 10% of Earth’s land surface (Figure 1), and offers a unique opportunity to study (almost, or as close to continues deposits we can get) continuous, terrestrial, Quaternary (and beyond [4]) paleoclimatic and paleoenvironmental change.
Loess sequences refer to alternating units of loess (yellowish dust) and palaeosols (brownish-reddish soil horizons (Figure 2 and image). Some locations preserve hundreds of meters of accumulation and over 30 loess-palaeosol packages. Loess units form during periods of peak dust accumulation, typically glacial and stadial periods. Loess can therefore be indicative of cold, arid, and windy climates with reduced vegetation and potentially increased sediment supply. Well-defined palaeosols are formed when the rate of pedogenesis (soil formation) exceeds the rate of dust accumulation. Thus, palaeosols typically develop during periods of landscape stability and under milder climatic conditions (warmer and/or wetter), and by extension during interglacial and interstadial periods. This cyclical manner not only provides a long-term, terrestrial view of Quaternary climatic cycles, but also a broad chronological framework. By counting the loess-palaeosol packages, one can relatively easily determine the Marine Isotopic Stage (MIS; numbered glacial and interglacial global conditions reflected in changes to the oxygen isotope content in marine cores) and therefore the time of deposition.
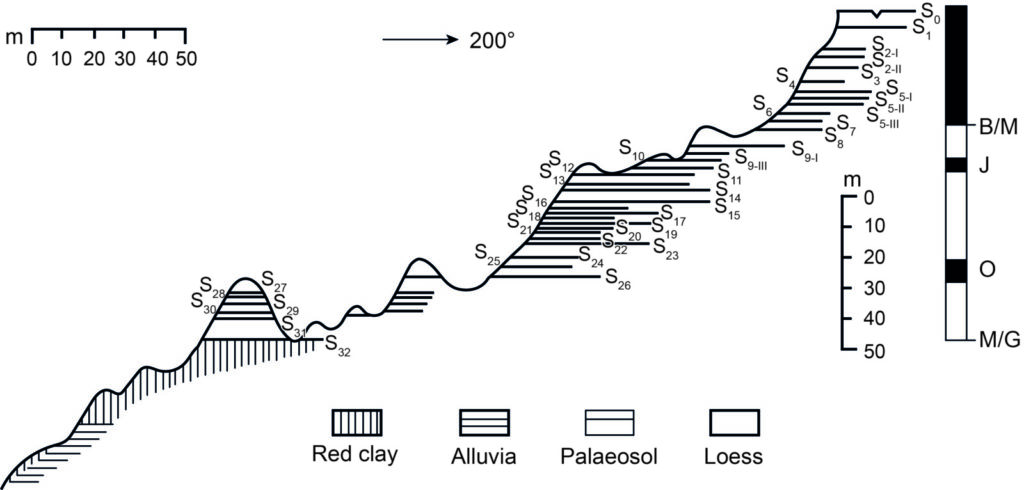
Figure 2. Loess-palaeosol units in Baoji (Chinese Loess Plateau) extending to approximately the Matuyama-Gauss magnetic boundary (2.58 Ma). Redrawn from [6].
When using loess as an archive of Quaternary change, sedimentary and pedological characteristics are the first analytical point, therefore let me summarise some of the fundamental aspects. Loess forms homogenous and massive (no bedding) sedimentary units, the thickness of which can vary from just a few centimetres to several meters. Sediment is dominated by silt size (4-63 μm) material, but is also poorly sorted and can contain sand (>63 μm) and clay (<4 μm) particles [7]. Variations in the proportion of grain size classes are typically used to reconstruct aeolian transport processes, such as wind strength, direction, and distance travelled. For example, an increased proportion of sand particles indicates stronger winds, and a more proximal sediment source. However, it could also point to reduced vegetation levels, potentially indicating a climatic deterioration. Other parameters (such as wind direction or sediment availability changes) may also play a role as well. Conversely, a higher proportion of clay particles suggests increased pedogenic processes (e.g. mineral breakdown) implying a warmer and more stable climate and landscape. However, clay particles can also be added to sections via long-range aeolian transport.
The interpretation of palaeosols imbedded within loess sequences (and by extension climatic, i.e. interglacial and interstadial periods) can be strengthened with magnetic susceptibility measurements. Chemical weathering of iron-bearing minerals during pedogenic processes produces a variety of iron oxides, such as maghemite, haematite and goethite, and results in a larger proportion of ferromagnetic minerals and a stronger magnetic signal in palaeosol than in loess. The iron-bearing minerals give soils a reddish-brownish-yellowish colour. Soil development is governed by climate, organisms, topography, parent material, and time, therefore identification of the soil type or pedogenic features can also be used to reconstruct past climatic and environmental conditions. For example, gleying (greenish-blue-grey colours) suggests periods of water-logging and low oxygen levels, and chernozem (dark-black, organic matter rich soils) indicates a steppe environment (grassland plains associated with semi-arid or continental climates). As soils develop into a loess unit (parent material remains constant), a shift in palaeosol type in a loess sequence can also be indicative of long-term climatic shifts.
Analyses of sedimentological proxies (e.g. sediment grain size and magnetic properties) and pedology have been the backbone of loess research for years. However, over the last three decades there has been an increasing number of geochemical and biological proxies utilised to strengthen and expand the interpretation. For example, molluscs (malacology) can be used to reconstruct habitat types and temperature ranges. Terrestrial molluscs are highly sensitive to environmental changes and are well preserved in carbonate loess sediments. They are also widely distributed geographically and have abundant modern analogues making them a reliable proxy. Additionally, being made of carbonate material, mollusc shells can be analysed for stable oxygen (δ18O) and carbon (δ13C) isotopes. The fractionation of δ13C and δ18O of soil carbonates can be used to reconstruct vegetation type (C3 and C4 plants), precipitation, and temperature [8–10]. Recent advances in organic geochemistry have resulted in the development of biomarker analysis, in particular the plant leaf-wax n-alkanes [11], which are able to further narrow down the vegetation types to e.g. herbs and grasses.
Inorganic geochemical analysis of loess sediments is often used to identify the strength of weathering and a loess profile’s pedogenic history [12–14]. Specific minerals, elements, and isotopic ratios are critical in establishing both sediment source and transport [13,15]. Additionally, the source can be reconstructed using individual mineral grains. As a single grain can only come from one source, these methods have been successful in identifying multi-source provenance signals as well as tracing continental-scale sediment transport and deposition dynamics [16,17]. Consequently, loess not only provides a variety of palaeoenvironmental and paleoclimatic information but is also geographically widespread and can span the length of the Quaternary period.
However, the abundance of proxies and detailed high-resolution reconstructions does not mean much if a time frame cannot be provided. The growing number geochronological approaches means that a range of proxies can be utilised to their best potential and terrestrial changes accurately placed in the context of climatic changes. As I mentioned before, a loess stratigraphy can be used as a geochronological guideline, however this means making two assumptions: (I) the deposit did not undergo erosion and (II) the deposit underwent a uniform response to climate. One could argue that erosive events capable of removing a whole loess or palaeosol unit (and creating an unconformity), would preserve evidence of such processes. However, the homogenous and massive nature of the units means that a new unit looks almost identical to the next in terms of its stratigraphy and sedimentology [18,19]. The uniform response to a climate also presents a challenge. For example, site specific variables such as topography and microclimate may result in two palaeosol horizons at one site and just one at a site several km away. Luckily, these assumptions only come into play for older units, which cannot be absolutely dated. On the Quaternary timescale, magnetostratigraphy (Figure 1) can be used to establish age tie-points and verify the age determined on the basis of stratigraphy. On a shorter timescale (e.g. last glacial-interglacial cycle), luminescence and radiocarbon dating are used to determine an absolute chronology, often supplemented with tephra markers [20,21]. Some studies based on sites on the Chinese Loess Plateau and Europe have been able to construct a millennial resolution chronologies [18,22,23]. Additionally, high-resolution chronological studies can be converted into a proxy dust flux and interpreted in a context of landscape activation and global dust archives.
I hope this overview of loess as an archive shows the incredible potential loess has for understanding Quaternary palaeo- environments and climates, both at orbital and sub-orbital scales. Though, I might be biased as a loess researcher!
This post has been edited by the editorial board.
References: [1] Pésci, M., 1990. Loess is not just the accumulation of dust. Quaternary International 7/8: 1–21, Doi: 10.1080/00288306.1964.10428132. [2] Wang, Y.J., Cheng, H., Edwards, R.L., An, Z., Wu, J.Y., Shen, C.C., et al., 2001. A high-resolution absolute-dated late pleistocene monsoon record from Hulu Cave, China. Science 294(5550): 2345–8, Doi: 10.1126/science.1064618. [3] Lacey, J.H., Leng, M.J., Francke, A., Sloane, H.J., Milodowski, A.E., Vogel, H., et al., 2016. Mediterranean climate since the Middle Pleistocene: A 640 ka stable isotope record from Lake Ohrid (Albania/Macedonia). Biogeosciences Discussions 12(16): 13427–81, Doi: 10.5194/bgd-12-13427-2015. [4] Guo, Z.T., Ruddiman, W.F., Hao, Q., Wu, H.B., Qiao, Y.S., Zhu, R.X., et al., 2002. Onset of Asian desertification by 22 Myr ago inferred from loess deposits in China. Nature 416(6877): 159–63, Doi: 10.1038/416159a. [5] Fenn, K., Prud’homme, C., 2021. Dust archives: Loess. In: Shroder, J.F., Lancaster, N., Sherman, D.J., Baas, A.C.W., editors. Treatise on Geomorphology, 2nd ed. Academic Press. [6] Ding, Z., Yu, Z., Rutter, N.W., Liu, T.-S., 1994. Towards an orbital time scale for chinese loess deposits. vol. 13. [7] Pye, K., 1984. Loess. Progress in Physical Geography 8(2): 176–217. [8] Gallant, C.E., Candy, I., van den Bogaard, P., Silva, B.N., Turner, E., 2014. Stable isotopic evidence for Middle Pleistocene environmental change from a loess-paleosol sequence: Kärlich, Germany. Boreas 43(4): 818–33, Doi: 10.1111/bor.12065. [9] Hatté, C., Gauthier, C., Rousseau, D.-D., Antoine, P., Fuchs, M., Lagroix, F., et al., 2013. Excursions to C 4 vegetation recorded in the upper pleistocene loess of surduk (Northern Serbia): An organic isotope geochemistry study. Climate of the Past 9(3): 1001–14, Doi: 10.5194/cp-9-1001-2013. [10] Prud’homme, C., Lécuyer, C., Antoine, P., Hatté, C., Moine, O., Fourel, F., et al., 2018. δ13C signal of earthworm calcite granules: A new proxy for palaeoprecipitation reconstructions during the Last Glacial in western Europe. Quaternary Science Reviews 179: 158–66, Doi: 10.1016/j.quascirev.2017.11.017. [11] Zech, R., Zech, M., Marković, S.B., Hambach, U., Huang, Y., 2013. Humid glacials, arid interglacials? Critical thoughts on pedogenesis and paleoclimate based on multi-proxy analyses of the loess-paleosol sequence Crvenka, Northern Serbia. Palaeogeography, Palaeoclimatology, Palaeoecology 387: 165–75, Doi: 10.1016/j.palaeo.2013.07.023. [12] Buggle, B., Glaser, B., Zöller, L., Hambach, U., Marković, S.B., Glaser, I., et al., 2008. Geochemical characterization and origin of Southeastern and Eastern European loesses (Serbia, Romania, Ukraine). Quaternary Science Reviews 27(9–10): 1058–75, Doi: 10.1016/j.quascirev.2008.01.018. [13] Skurzyński, J., Jary, Z., Raczyk, J., Moska, P., Korabiewski, B., Ryzner, K., et al., 2019. Geochemical characterization of the Late Pleistocene loess-palaeosol sequence in Tyszowce (Sokal Plateau-Ridge, SE Poland). Quaternary International 502(March 2018): 108–18, Doi: 10.1016/j.quaint.2018.04.023. [14] Guan, H., Zhu, C., Zhu, T., Wu, L., Li, Y., 2016. Grain size, magnetic susceptibility and geochemical characteristics of the loess in the Chaohu lake basin: Implications for the origin, palaeoclimatic change and provenance. Journal of Asian Earth Sciences 117: 170–83, Doi: 10.1016/j.jseaes.2015.12.013. [15] Campodonico, V.A., Rouzaut, S., Pasquini, A.I., 2019. Geochemistry of a Late Quaternary loess-paleosol sequence in central Argentina: Implications for weathering, sedimentary recycling and provenance. Geoderma 351(December 2018): 235–49, Doi: 10.1016/j.geoderma.2019.04.024. [16] Nie, J., Stevens, T., Rittner, M., Stockli, D.F., Garzanti, E., Limonta, M., et al., 2015. Loess Plateau storage of Northeastern Tibetan Plateau-derived Yellow River sediment. Nature Communications 6, Doi: 10.1038/ncomms9511. [17] Stevens, T., Carter, A., Watson, T.P., Vermeesch, P., Andò, S., Bird, A.F., et al., 2013. Genetic linkage between the Yellow River, the Mu Us desert and the Chinese Loess Plateau. Quaternary Science Reviews 78: 355–68, Doi: 10.1016/j.quascirev.2012.11.032. [18] Stevens, T., Buylaert, J.-P., Thiel, C., Újvári, G., Yi, S., Murray, A.S., et al., 2018. Ice-volume-forced erosion of the Chinese Loess Plateau global Quaternary stratotype site. Nature Communications 9(1): 983, Doi: 10.1038/s41467-018-03329-2. [19] Fenn, K., Durcan, J.A., Thomas, D.S.G., Banak, A., 2020. A 180 ka record of environmental change at Erdut (Croatia): a new chronology for the loess–palaeosol sequence and its implications for environmental interpretation. Journal of Quaternary Science 35(4): 582–93, Doi: 10.1002/jqs.3201. [20] Fitzsimmons, K.E., Hambach, U., 2014. Loess accumulation during the last glacial maximum: Evidence from Urluia, southeastern Romania. Quaternary International 334–335: 74–85, Doi: 10.1016/j.quaint.2013.08.005. [21] Jensen, B.J.L., Evans, M.E., Froese, D.G., Kravchinsky, V.A., 2016. 150,000 years of loess accumulation in central Alaska. Quaternary Science Reviews 135: 1–23, Doi: 10.1016/j.quascirev.2016.01.001. [22] Újvári, G., Stevens, T., Molnár, M., Demény, A., Lambert, F., Varga, G., et al., 2017. Coupled European and Greenland last glacial dust activity driven by North Atlantic climate. Proceedings of the National Academy of Sciences: 201712651, Doi: 10.1073/pnas.1712651114. [23] Moine, O., Antoine, P., Hatté, C., Landais, A., Mathieu, J., Prud’homme, C., et al., 2017. The impact of Last Glacial climate variability in west-European loess revealed by radiocarbon dating of fossil earthworm granules. Proceedings of the National Academy of Sciences 114(24): 6209–14, Doi: 10.1073/pnas.1614751114.
Ian James SMALLEY
Always good to read about Loess; try the ‘Loess Ground’ blog (www.loessground.blogspot.com).
Denis-Didier Rousseau
2017 may be very far, but my Oeschger medal lecture at EGU GA was amazingly titled “(MIS3 & 2) millennial oscillations in Greenland dust and Eurasian aeolian records – a Paleosol-Loess perspective”…:-)
Sam skidmore
Our loess sequence is similar separated by alternating warm climate gravel beds. Holocene through Miocene sediments lay above Precambrian schist bedrock highly decomposed below a Pliocene gravel bed.