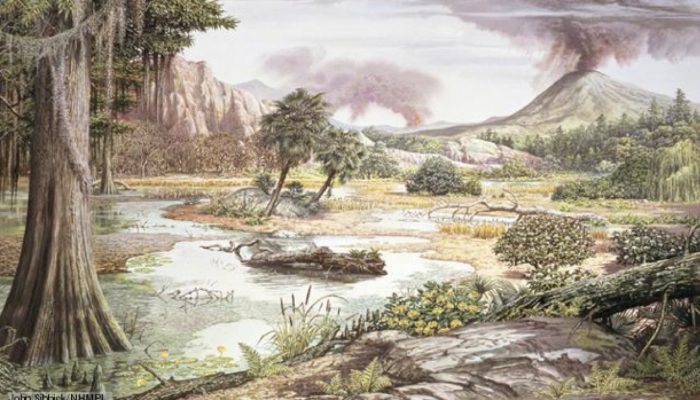
The Cretaceous
The Cretaceous period features a particularly interesting climatic episode in the Earth’s geological history. It follows the Jurassic Period, better known as the time the dinosaurs inhabited Earth and spanned the period between 145.5 and 65.5 million years ago. The Cretaceous is the last period of the Mesozoic Era, which ends with a well-known mass extinction event. At the end of the Cretaceous, an asteroid hit the Earth in the Yucatan Peninsula, Mexico, forming what is today called the Chicxulub impact crater (2). It has been estimated that half of the world’s species became extinct around this time, but no accurate species count exists for each group of organisms. Figure 1 represents an artistic representation of how we imagine the Cretaceous landscape looked like.The supercontinent Pangea, which already started to rift apart in the preceding period, continued to diverge. By the mid-Cretaceous, Pangea split into several smaller continents and ocean basins (continental configuration illustrated in Figure 2), such as the Pacific Ocean, the proto-Atlantic and the Tethys Sea. The spreading of continents also generated extensive new coastlines resulting in increasing near-shore habitats. Moreover, seasons became more pronounced as the global climate cooled. By the end of the Cretaceous, primordial woods evolved to become more similar to those distributed on Earth today (2).
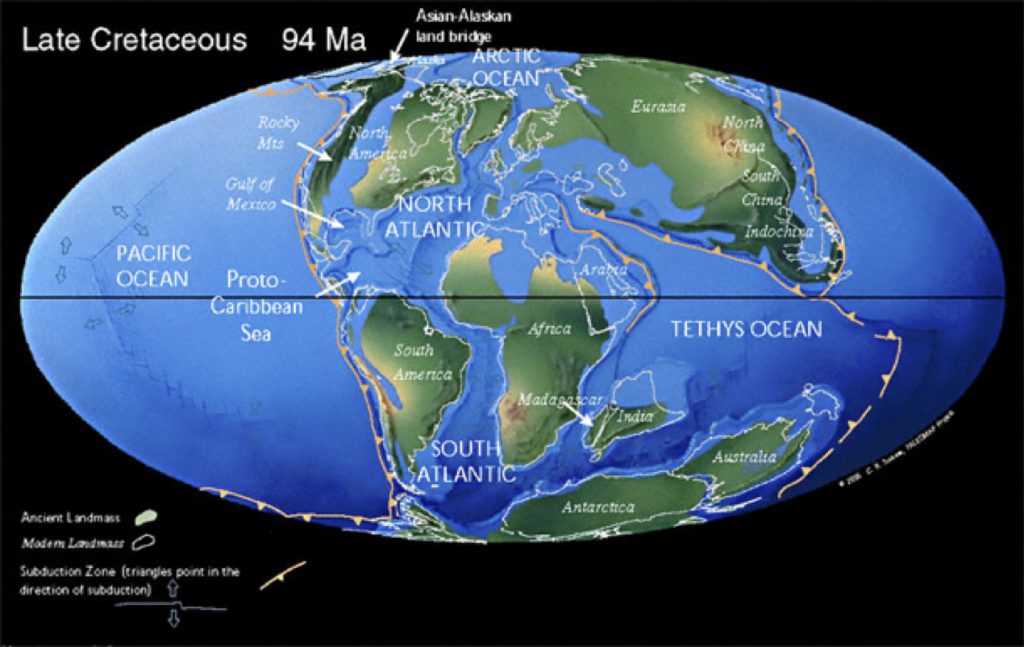
Figure 2: Much of today’s dry land – most of Europe, the midwest of the USA and Northern Africa- was underwater, due to high sea levels during the Cretaceous. The proto-Atlantic Ocean grew much wider as North and South America rifted apart from Africa and Europe. The Indian continent was still an island drifting northward to encounter the Asian continent. (Source: http://www.scotese.com/cretaceo.htm, visited 14.7.2018)
A stable and warm climate
Another intriguing aspect of the Cretaceous period is the warm and stable climate, with tropical and polar temperatures higher than today, lower gradient from the Equator to the Poles, as well as from the land to the ocean and fewer seasonal extremes. Rainfall and atmospheric greenhouse gas concentrations (e.g. CO2, CH4) were higher in the Cretaceous compared to today explaining partly the relatively warmer climate at the global scale. High temperatures extended into the polar regions have prevented the accumulation of ice sheets and reduced the temperature gradient between the Equator and the Poles. This in turn disrupted the mid- and high latitude wind systems, which affected global temperature distribution and the wind-driven ocean circulation (2,5).
The lack of continuous winds at mid-latitudes prevented ocean currents from forming and transporting heat from the Equator to higher latitudes. In today’s ocean, the Gulf Stream would be an example of such a current, able to transport heat from the Caribbean area all the way to Europe, making winter months in Europe warmer. During the Cretaceous this heat was transported via small to large scale swirls capable to trap heat and transport it to higher latitudes. The dominating “thermohaline circulation”, i.e. the current global ocean circulation, would not develop until the continental configuration was similar to todays.
The Cretaceous Ocean and its chemical state
The chemical state of the Cretaceous ocean was also extremely different. Today, oceans are dominated by oxic (oxygenated) water masses, with the exception of some marginal basins like the Black Sea, fjords, upwelling areas and so-called coastal “anoxic dead zones”. However, during the Jurassic and early Cretaceous episodes local anoxia occurred and this state developed into a global scale phenomenon during the mid-Cretaceous. These Oceanic Anoxic Events (OAEs) represented severe disturbances to the global carbon, oxygen and nutrient cycles of the ocean. One well-known event is the OAE2, which happened during the mid-Cretaceous (120-80 Ma), shown in Figure 3 together with several other OAEs recorded in a sediment core drilled in Northern Germany. The OAE2 was characterized by extreme atmospheric CO2 concentrations, widespread water anoxia and free hydrogen sulfide in the surface ocean (3). Evidence for these global perturbations is recorded in ocean sediments, thus providing an important insight into the Earth’s climate archive. Sediments from this period attributed to OAE2 are known globally for their thick layers of organic-rich black shales. The enhanced accumulation of organic carbon during these extreme events did not only have a significant impact on Cretaceous ocean chemistry and climate, but also played an important role in the formation of oil and gas, over millions of years, which are now extensively exploited as a fossil energy source (4).
The Cretaceous Black Shale
The resulting elevated levels of carbon burial would account for the Cretaceous Black Shale Formation in the ocean basins and has often been related to increased organic matter preservation due to anoxic conditions, increased oceanic productivity in the surface ocean or a combination of both. However, the exact nature and background of the paleo-environment that fostered this massive and almost global deposition of organic carbon-rich sediments is still a matter of debate. Two mechanisms are commonly proposed to explain the prolonged oceanic oxygen depletion during OAEs: first, a decreased oxygen supply to the deep ocean due to weaker ocean circulation and second, an increased oxygen demand in the water column resulting from enhanced primary productivity (1,4).
In the latter mechanism, the high primary production was caused by the increased nutrient availability in the ocean in combination with high temperatures. This has been shown by following the evolution of the nutrient concentration in sediments from the onset of an OAE to the end of it: Nutrients (phosphorous in this case) accumulate in the sediments prior to an OAE, but their concentrations decrease during an anoxic event. This implies that the nutrients were consumed by primary producers (4). As primary producers die and sink as organic matter through the low oxygen concentration water column, one part of the organic matter is degraded, while the other part reaches the ocean floor, where it is buried layer upon layer, creating thick sediment bands with high concentrations of organic matter. These layers show up as dark laminated layer in sediment cores (Figure 3).
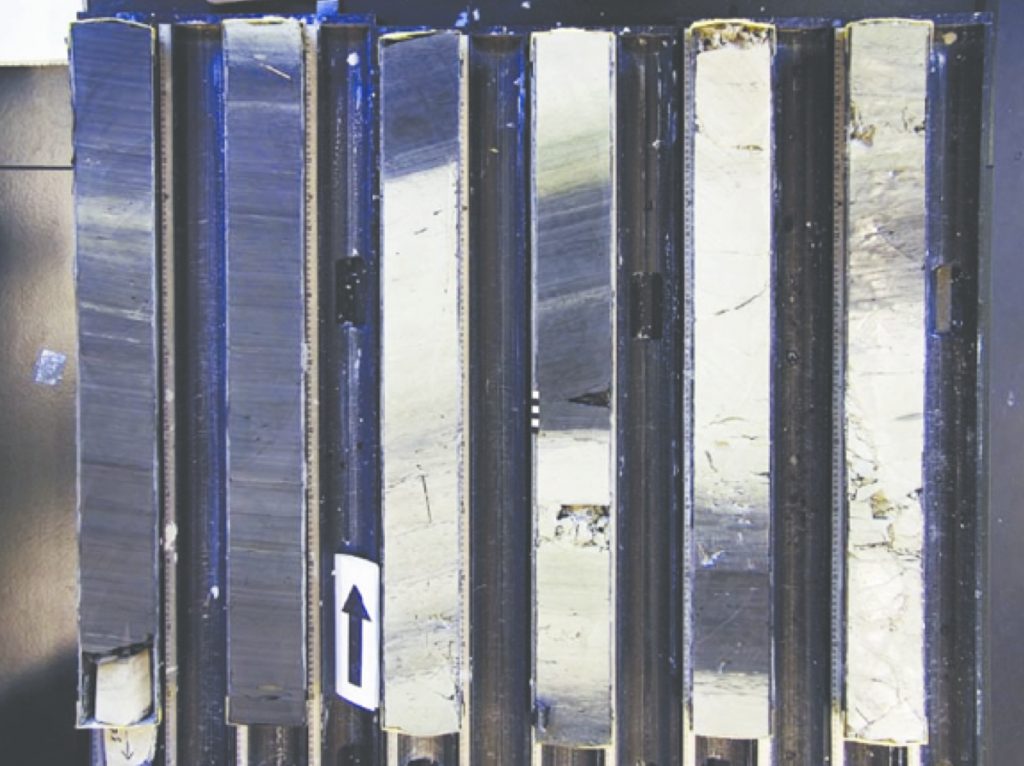
Figure 3: The history of Earth can be read from this sediment core. Several transitions between an oxic and anoxic states are visible in this core section (meters 44 to 38 of the Wunstorf core), whereby the black shales represented by the dark laminated layers. This sediment core was taken on land in Northern Germany during the time when this region was under water (mid Cretaceous).
(Source: https://www.researchgate.net/publication/49595125/download, visited 14.7.2018)
These black shales strata offer a unique opportunity to study the evolution of biological, chemical and physical processes in sediments. It allows us to investigate the relation between sediment accumulation and the degradation of organic matter over the geological times. This can bring key information on the evolution of carbon sources and sinks and their possible climate feedback on different time scales.
This post has been edited by the editorial board.
References
-
Arndt, Sandra, Hans-Jürgen Brumsack, and Kai W. Wirtz. "Cretaceous black shales as active bioreactors: a biogeochemical model for the deep biosphere encountered during ODP Leg 207 (Demerara Rise)." Geochimica et Cosmochimica Acta 70.2 (2006): 408-425.
-
Harff, Jan, et al. Encyclopedia of Marine Geosciences. Springer, 2016.
-
Hay, William W. "Evolving ideas about the Cretaceous climate and ocean circulation." Cretaceous Research 29.5-6 (2008): 725-753.
-
Hülse, Dominik, et al. "Understanding the causes and consequences of past marine carbon cycling variability through models." Earth-science reviews 171 (2017): 349-382.
-
Weissert, Helmut. "Mesozoic pelagic sediments: archives for ocean and climate history during green-house conditions." Developments in sedimentology. Vol. 63. Elsevier, (2011): 765-792.
Dice View
Very helpful and Great information,
we appreciate advise especially coming from a professional.
Thanks again and keep up the great work!