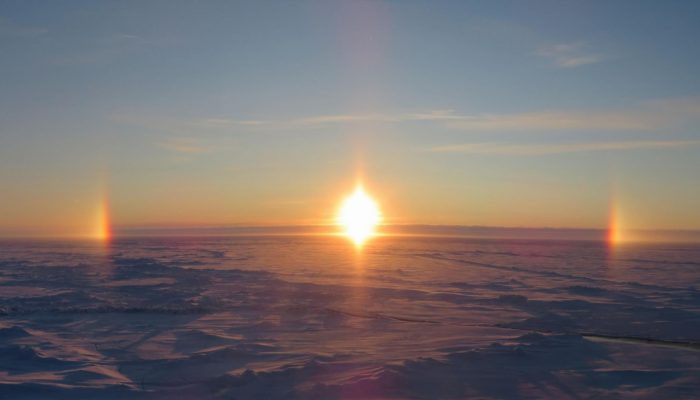
Current Position: 86°24’ N, 13°29’E (17th September 2018)
The Arctic Ocean 2018 Expedition drifted for 33 days in the high Arctic and is now heading back south to Tromsø, Norway. With continuous aerosol observations, we hope to be able to add new pieces to the high Arctic aerosol puzzle to create a more complete picture that can help us to improve our understanding of the surface energy budget in the region.
In recent years, considerable efforts have been undertaken to study Arctic aerosol. However, there are many facets to Arctic aerosol so that different kinds of study designs are necessary to capture the full picture. Just to name a few efforts, during the International Polar Year in 2008, flight campaigns over the North American and western European Arctic studied the northward transport of pollution plumes in spring and summer time [1,2,3]. More survey-oriented flights (PAMARCMIP) have been carried out over several years and seasons [4] around the western Arctic coasts. The NETCARE campaigns [5] have studied summertime Canadian Arctic aerosol in the marginal ice zone. And the Arctic Monitoring and Assessment Programme (AMAP) has issued reports on the radiative forcing of anthropogenic aerosol in the Arctic [6,7].
These and many other studies have advanced our understanding of Arctic aerosol substantially. Since the 1950s we are aware of the Arctic Haze phenomenon that describes the accumulation of air pollution in the Arctic emitted from high latitude sources during winter and early spring. In these seasons, the Arctic atmosphere is very stratified, air masses are trapped under the so-called polar dome and atmospheric cleansing processes are minimal. In springtime, with sunlight, when the Arctic atmosphere becomes more dynamic, the Arctic Haze dissolves with air mass movement and precipitation. Then, long-range transport from the mid-latitudes can be a source of Arctic aerosol. This includes anthropogenic as well as forest fire emissions. The latest AMAP assessment report [6] has estimated that the direct radiative forcing of current global black and organic carbon as well as sulfur emissions leads to a total Arctic equilibrium surface temperature response of 0.35 °C. While black carbon has a warming effect, organic carbon and particulate sulfate cool. Hence, over the past decades the reductions in sulfur emissions from Europe and North America have led to less cooling from air pollution in the Arctic [8]. Currently, much effort is invested in understanding new Arctic emission sources that might contribute to the black carbon burden in the future, for example from oil and gas facilities or shipping [9, 10, 11].
These studies contribute to a more thorough understanding of direct radiative effects from anthropogenic aerosol and fire emissions transported to the Arctic. However, neither long-range transported aerosol nor emissions within the lower Arctic contribute substantially to the aerosol found in the boundary layer of the high Arctic [12]. These particles are emitted in locations with warmer temperatures and these air masses travel north along isentropes that rise in altitude the further north they go. The high Arctic boundary layer aerosol, however, is important because it modulates the radiative properties of the persistent Arctic low-level clouds that are decisive for the surface energy budget (see first Arctic Ocean blog in August 2018).
Currently, knowledge about sources and properties of high Arctic aerosol as well as their interactions with clouds is very limited, mainly because observations in the high Arctic are very rare. In principle, there are four main processes that shape the aerosol population in the high north: a) primary sea spray aerosol production from open water areas including open leads in the pack ice area, b) new particle formation, c) horizontal and vertical transport of natural and anthropogenic particles, and d) resuspension of particles from the snow and ice surface (snowflakes, frost flowers etc.). From previous studies, especially in the marginal ice zone and land-based Arctic observatories, we know that microbial emissions of dimethyl sulfide and volatile organic compounds are an important source of secondary aerosol species such as particulate sulfate or organics [13]. The marginal ice zone has also been identified as potential source region for new particle formation [14]. What is not known is to which degree these particles are transported further north. Several scavenging processes can occur during transport. These include coagulation of smaller particles to form larger particles, loss of smaller particles during cloud processing, precipitation of particles that acted as cloud condensation nuclei or ice nucleating particles, or sedimentation of large particles to the surface.
Further north in the pack ice, the biological activity is thought to be different compared to the marginal ice zone, because it is limited by the availability of nutrients and light under the ice. Hence, local natural emissions in the high Arctic are expected to be lower. Similarly, since open water areas are smaller, the contribution of primary marine aerosol is expected to be lower. In addition, the sources of compounds for new particle formation that far north are not very well researched.
To understand some of these sources and their relevance to cloud properties, an international team is currently measuring the aerosol chemical and microphysical properties in detail during the Arctic Ocean 2018 expedition on board the Swedish icebreaker Oden. It is the fifth expedition in a series of high Arctic field campaigns on the same icebreaker. Previous campaigns took place in 1991, 1996, 2001 and 2008 (see refs [15, 16, 17, 18] and references therein).
The picture below describes the various types of air inlets and cloud probes that are used to sample ambient aerosol particles and cloud droplets or ice crystals. A large suite of instrumentation is used to determine in high detail the particle number concentrations and size distribution of particles in the diameter range between 2 nm and 20 µm. Several aerosol mass spectrometers help us to identify the chemical composition of particles between 15 nm and 1 µm as well as the clusters and ions that contribute to new particle formation. Filter samples of particles smaller than 10 µm will allow a detailed determination of chemical components of coarse particles. They will also give a visual impression of the nature of particles through electron microscopy. Filter samples are also used for the determination of ice nucleation particles at different temperatures. Cloud condensation nuclei counters provide information on the ability of particles to form cloud droplets. A multi-parameter bioaerosol spectrometer measures the number, shape and fluorescence of particles. Further instruments such as black carbon and gas monitors help us to distinguish pristine air masses from long-range pollution transport as well as from the influence of the ship exhaust. We can distinguish and characterize the particle populations that do or do not influence low-level Arctic clouds and fogs in detail by using three different inlets: i) a total inlet, which samples all aerosol particles and cloud droplets/ice crystals, ii) an interstitial inlet, which selectively samples particles that do not form droplets when we are situated inside fog or clouds, and iii) a counterflow virtual impactor inlet (CVI), which samples only cloud droplets or ice crystals (neglecting non-activated aerosol particles). The cloud droplets or ice crystals sampled by the CVI inlet are then dried and thus only the cloud residuals (or cloud condensation nuclei) are characterized in the laboratory situated below.
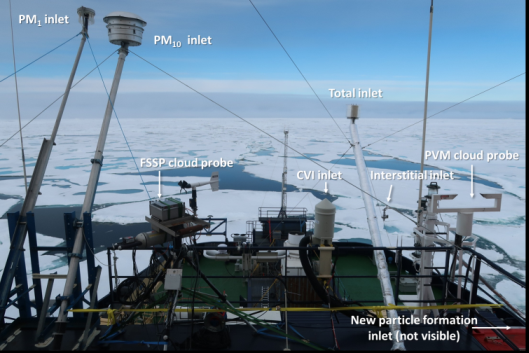
Inlet and cloud probe set-up for aerosol and droplet measurements installed on the 4th deck on board the icebreaker Oden. From left to right: Inlets for particulate matter smaller 1 µm (PM1) and smaller 10 µm (PM10); forward scattering spectrometer probe (FSSP) for droplet size distribution measurements; counterflow virtual impactor inlet (CVI) for sampling cloud droplets and ice crystals; total inlet for sampling of all aerosol particles and cloud droplets/ice crystals; interstitial inlet for sampling non-activated particles; particle volume monitor (PVM) for the determination of cloud liquid water content and effective droplet radius. Newly formed , very small, particles are sampled with a different inlet (not shown in the picture) specifically designed to minimize diffusion losses. (Picture credit: Paul Zieger)
To gain more knowledge about the chemical composition and ice nucleating activity of particles in clouds, we also collect cloud and fog water on the uppermost deck of the ship and from clouds further aloft by using tethered balloon systems. When doing vertical profiles with two tethered balloons, also particle number concentration and size distribution information are obtained to understand in how far the boundary layer aerosol is mixed with the cloud level aerosol. Furthermore, a floating aerosol chamber is operated at an open lead near the ship to measure the fluxes of particles from the water to the atmosphere. It is still unknown whether open leads are a significant source of particles. For more details on the general set-up of the expedition see the first two blogs of the Arctic Ocean Expedition (here and here).
After 33 days of continuous measurements while drifting with the ice floe and after having experienced the partial freeze-up of the melt ponds and open water areas, it is now time for the expedition to head back south. We will use two stations in the marginal ice zone during the transit into and out of the pack ice as benchmarks for Arctic aerosol characteristics south of our 5-week ice floe station.
As Oden is working her way back through the ice and the expedition comes to an end, we recapitulate what we have measured in the past weeks. What was striking, especially for those who have spent already several summers in the pack ice, is that this time the weather was very variable. There were hardly two days in row with stable conditions. Instead, one low pressure system after the other passed over us, skies changed from bright blue to pale grey, calm winds to storms… On average, we have experienced the same number of days with fog, clouds and sunshine as previous expeditions, but the rhythm was clearly different. From an aerosol perspective these conditions meant that we were able to sample a wide variety characteristics including new particle formation, absence of cloud condensation nuclei with total number concentrations as low as 2 particles per cubic centimeter, coarse mode particles, and size distributions with a Hoppel-minimum that is typical for cloud processed particles.
Coming back home, we can hardly await to fully exploit our recorded datasets. Stay tuned!
Do not hesitate to contact us for any question regarding the expedition and measurements. Check out this blog for more details of life during the expedition and our project website which is part of the Arctic Ocean 2018 expedition.
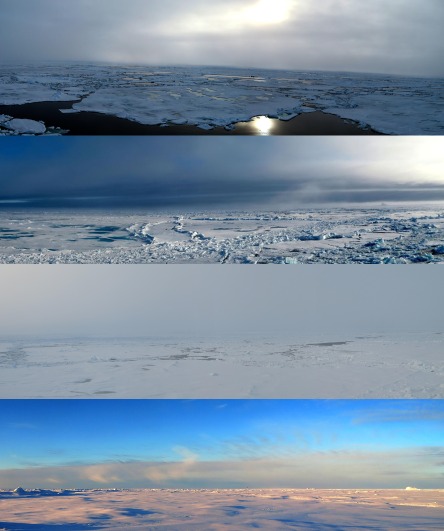
Changing Arctic landscapes. From top to bottom: Upon arrival at the drift station there were many open leads. Storms pushed the floes together and partially closed leads. Mild and misty weather. Cold days and sunshine lead to freeze-up. (Credit: Julia Schmale)
Edited by Dasaraden Mauree
Julia Schmale is a scientist in the Laboratory of Atmospheric Chemistry at the Paul Scherrer Institute, Switzerland. She has been involved in Arctic aerosol research for the past 10 years.
Andrea Baccarini, is doing his PhD in the Laboratory of Atmospheric Chemistry at the Paul Scherrer Institute, Switzerland. He specializes in new particle formation in polar regions.
Paul Zieger, is an Assistant Professor in Atmospheric Sciences at the University of Stockholm, Sweden. He is specialized in experimental techniques for studying atmospheric aerosols and clouds at high latitudes.
Latest Technology
Thanks for sharing this information.Very interesting.