Radiocarbon dating is probably the most well-known chronological technique regularly employed by archaeologists, geomorphologists and researchers analysing sediment cores. Palaeolimnologists (those who study lake sediments) hoping to determine the age of a sample extracted from a long sediment core traditionally seek terrestrial plant macrofossils (fragments of organic matter visible without a microscope), as these are considered most likely to return a reliable date. When I was searching for material to send to the radiocarbon lab during my PhD, finding bits of leaf from a tree, seeds or twigs made me especially excited.
A new paper written by James Marty and Amy Myrbol (Department of Earth Sciences, University of Minnesota) and published in Journal of Paleolimnology (MM2014) reviews the feasibility of acquiring robust ages from certain aquatic plant macrofossils. It was an interesting read, as I had not previously considered their viability, and I believe worthy of attention from the palaeolimnology community. The online Tool for Macroscopic Identification available from LacCore team at the University of Minnesota is a valuable resource too.
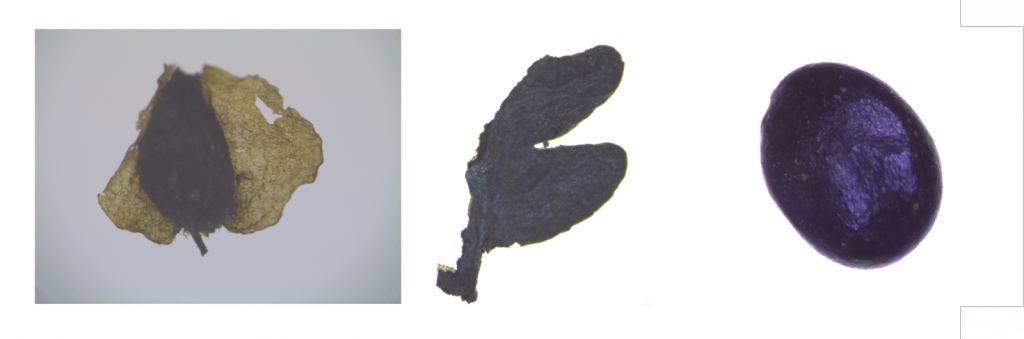
Examples of terrestrial plant macrofossils found in a sediment core extracted from Brotherswater, England. From left to right: Birch seed; leaf fragment; alder seed. Photos: D. Schillereff.
How does radiocarbon dating work?
Aquatic plants have traditionally been considered poor recorders of atmospheric carbon-14 concentrations, which is the critical bit of information we need to calculate an age; we’ll take a look at the mechanics of radiocarbon dating to understand why. If you’re interested, there are loads of useful websites that provide more details of radiocarbon dating or check out this video from Scientific American:
Because 14C is continuously formed in the atmosphere, the amount of 14C in the living tissue of the terrestrial plant is in equilibrium with atmospheric levels for as long as the plant is alive, photosynthesising and thus exchanging carbon.
Eventually the plant dies and a fragment, perhaps a leaf, breaks off and is blown or floats down a river to the lake, where it sinks to the bottom and is buried by other sediment. The atmospheric concentration of 14C is ‘locked’ in the tissue of the plant fragment at the time of its death – effectively ‘starting the clock’. The carbon-14 isotope is unstable and decays at a known rate, or half-life; in other words, the ‘ticking of the clock’. The half-life is the length of time it takes for 50% of the 14C atoms to decay and be lost; in the case of carbon-14 this is 5730 years. By measuring how much 14C remains in the plant material today, we can work backwards and calculate how many years ago the plant must have died (within a window of error).
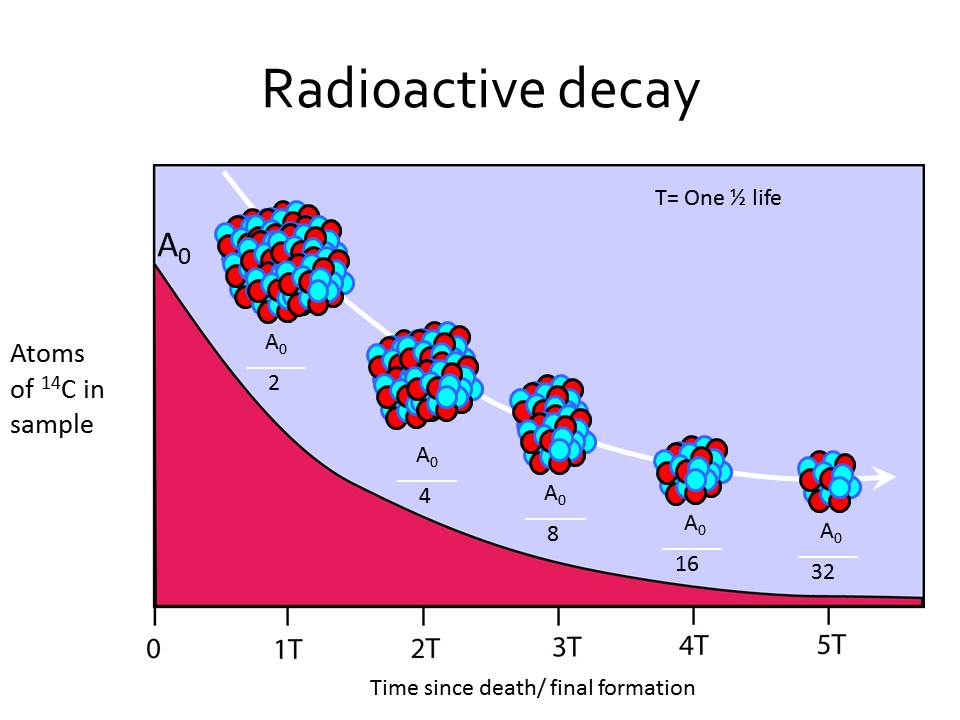
Schematic illustrating the radioactive decay process of 14C atoms. Diagram courtesy of P. Ascough, SUERC
What about aquatic plants?
A major problem with attempting to date the remains of an aquatic plant is that many species absorb dissolved inorganic carbon (DIC) from the surrounding water column. Unlike atmospheric carbon, DIC may originate from carbonate rocks that precipitate into the water; carbon from this source is geologically ancient and not reflective of contemporaneous atmospheric concentrations. An attempt to calculate a radiocarbon date would probably yield an age much older (potentially thousands of years) than the sediment layer in which the plant fragment was found. This introduction of an artificially old age is known as the ‘reservoir effect’.
Assessing the comparative uptake of atmospheric carbon (good for dating) versus DIC on a species-specific basis is therefore critical but non-trivial task. Much of the MM2014 paper describes the physiology of different aquatic plants in terms of their mechanisms for absorbing atmospheric or dissolved, aqueous, inorganic carbon, and they conclude that, with few exceptions, submerged plants (roots and shoots entirely underwater) should be avoided. On the other hand, many emergent plants (defined by MM2014 as those “that may experience both atmospheric and aquatic environments under normal conditions”), have similar leaf structures to terrestrial plants and certainly exchange gases with the atmosphere. Other species have sub-surface leaves that enhance DIC uptake from the water column or root systems that absorb dissolved CO2 from the sediment, however, so a trained eye is required to identify a plant to species-level from a macrofossil.
MM2014 do highlight some case studies where reliable 14C dates were returned from emergent aquatic macrofossils. They also include a table summarising the mechanisms of DIC uptake and estimated rates for 35 different species. I was surprised to read that the likelihood for DIC fixation remains unknown for the majority of species, which is clearly a significant gap in plant physiology that would be valuable to address for palaeolimnologists.
As a brief conclusion, this paper is quite timely for me as I’m planning to undertake further sub-sampling for radiocarbon dates before Christmas so I will spend some time trawling through their website lists for potential candidates.