Bouâmama Cauliflowers (Fredolia aretioides) in the stony plateaus of the Eastern High Atlas near Ayt Wazag (Morocco). The compact, cushion-like habit, the tiny coriaceous leaves and the deep-reaching roots of this shrub allow it to thrive under arid climatic conditions. Photo by Luca Barale, as described on imaggeo.egu.eu. Imaggeo is the EGU’s online open access geosciences image repository ...[Read More]
Imaggeo On Monday: The desert of the cauliflowers
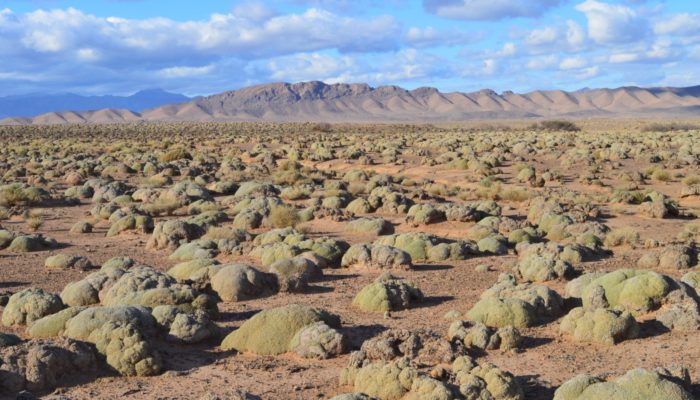