“The ocean is not too big to fail, nor is it too big to fix, but it is too important to ignore.” Last week, Dr Jane Lubchenco voiced a thought we’ve all had at some point: that the ocean is so vast and bountiful, it is almost inconceivable that people could impact it. And yet… Speaking to more than 20 Heads of State and Government, and an audience of thousands of policymakers, ocean scientists, bu ...[Read More]
An ocean emergency is upon us. Can the world reverse ocean decline?
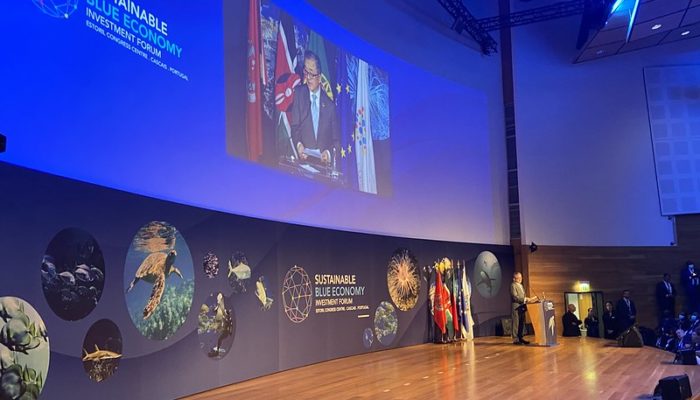