Disclaimer: This article contains minor spoilers for Season 8 of “Game of Thrones.” A basic understanding of the world of Game of Thrones is assumed in this post. The Game of Thrones world of ice and fire is an unpredictable place both politically and environmentally. While the fate of the Iron Throne is yet to be confirmed, a humble steward has been working diligently to make some sense of the pl ...[Read More]
Geosciences Column: climate modelling the world of Game of Thrones
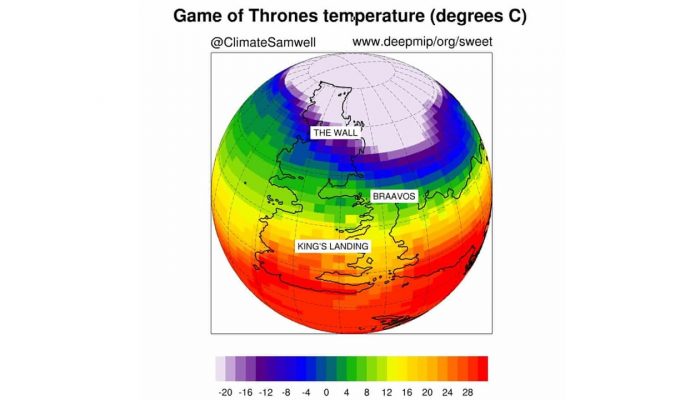