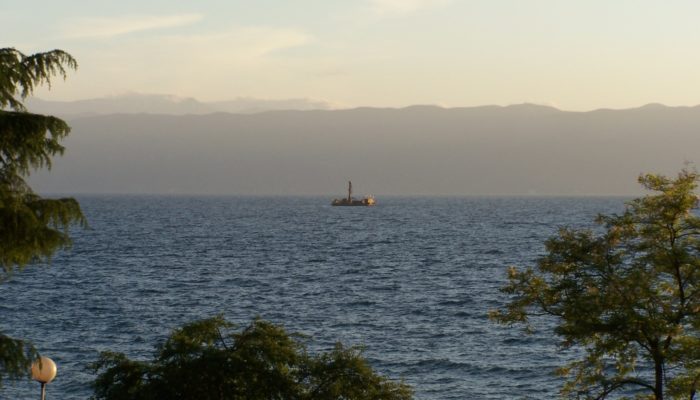
What is half-precession?
In the first half of the 20th century, the Serbian researcher Milutin Milanković described the periodic changes of the Earth’s orbit around the Sun. In recent decades, changes in these Milanković cycles have been shown to have an impact on global climate, and information about this is preserved in geologic records through sedimentological processes. Today we can study these sedimentary rocks and derive conclusions about paleoclimatic conditions. The ‘classical’ Milanković cycles play an important role in this context and consist primarily of three components: Eccentricity, the circular shape of the Earth’s orbit around the Sun; Obliquity, the tilt of the Earth’s axis; and Precession, which is the direction of the tilt of the Earth’s axis relative to the Sun.
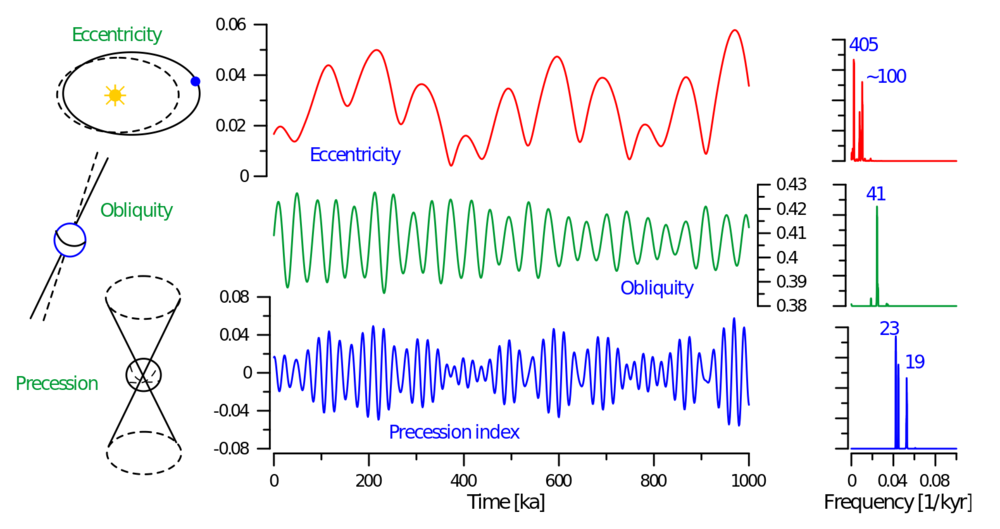
Figure 1: Geometry of the ‘classical’ Milanković cycles eccentricity, obliquity and precession (left), evolution of Milanković cycles over the last 1 Ma in the time-domain (center) and frequency domain (right). The main periodic components are given in thousand years (kyr) in the frequency domain. Figure from https://www.cyclostratigraphy.org
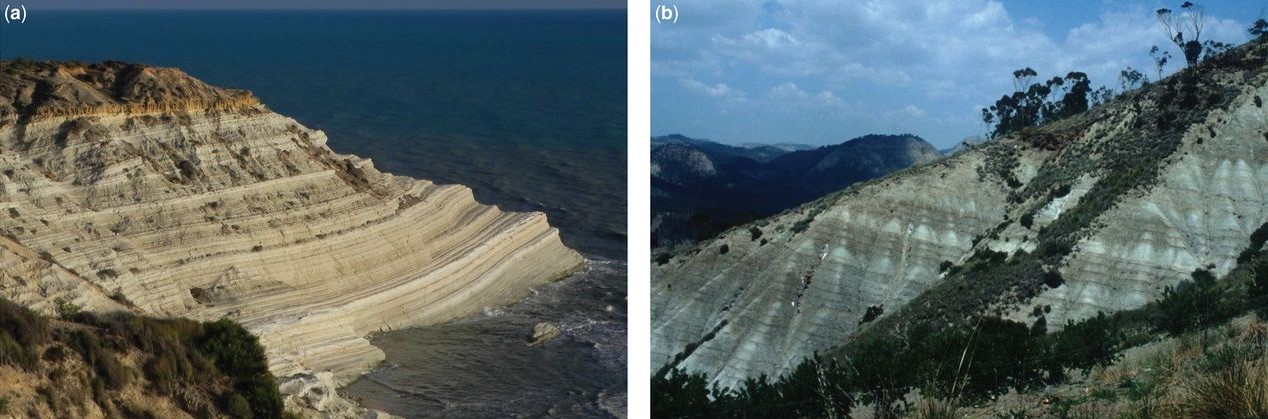
Figure 2: Examples of cyclicities in rock formations from marine environments exposed on Sicily (Italy) in Punta di Maiata (a) and Monte Gibliscemi (b). In comparison to the material shown in these photos, the material we investigate consists of soft sediments from the bottom of a lake (from Hilgen et al. 2015).
There are countless publications about these ‘classical’ Milanković cycles using various different proxies from marine-, terrestrial- and ice-environments. However, only a few studies describe half-precession, and most of them focus und (sub-) tropical records because the presumed origin of the signal is located there. But what is half-precession and why could it be of importance for Europe, or in general for mid- to high latitudes?
As the name implies, half-precession is half a precession cycle and thus has a duration of ~ 12.000-9.000 years. It is the result of two insolation maxima during one precession cycle in the tropical regions. An analogue would be the passage of the sun above the equator twice per year during the so called vernal and autumn equinoxes. During these times, the sun is directly above the equator and the day and night are exactly the same length in the northern and southern hemispheres. Thus, during one year there are two insolation maxima at the equator. According to a similar principle, during one precession cycle, two insolation maxima occur at the equator and generate the half-precession signal.
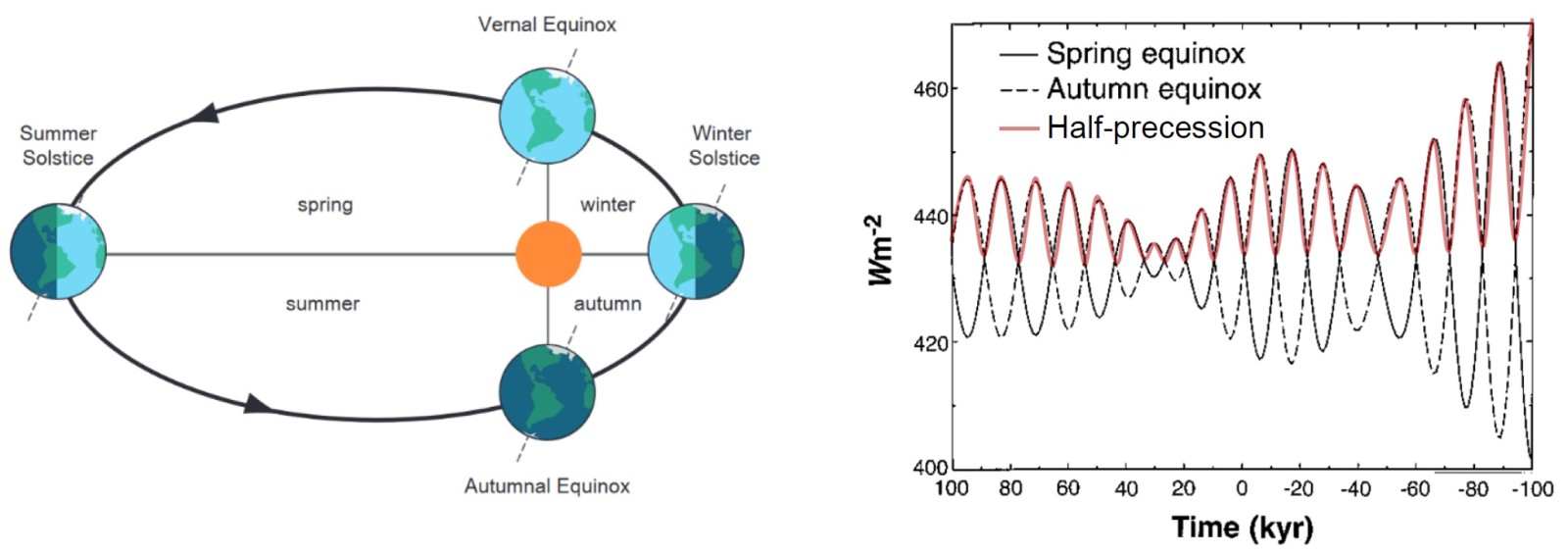
Figure 3: Left panel: Earth’s orbit including the winter- and summer solstices. The equinoxes happen twice per year. These are the days when the Sun is exactly above the Equator, causing 12 hours of daylight and 12 hours of night everywhere on Earth (from https://www.cyclostratigraphy.org). Right panel: Equatorial insolation at the spring- and autumn equinoxes. Note the insolation maximum every half-cycle (modified from Berger and Loutre, 1997)
The location
There is this lake on the Balkan Peninsula called Lake Ohrid. It is located in a basin on the border between Albania and North Macedonia, extends approximately 30 km from north to south, is 15 km wide and has a maximum depth of 293 m. The main contributor of water input to Lake Ohrid is a karst system fed by Lake Prespa in the east. The karst water flows through the Galičica Mountains, which consist of carbonates, thus delivering dissolved carbonate to Lake Ohrid. This process is enhanced in interglacials, i.e. warmer periods of the global climate, and is reflected in the sediment layers deposited in the lake.
Lake Ohrid is a very old lake that has existed for more than 1.36 Ma, which means that there was (and is) continuous sedimentation since 1.36 Ma. In 2013, the International Continental Scientific Drilling Program (ICDP) recovered a long sediment core from the central part of the lake. Since then, several publications have been released about this record and Lake Ohrid in general, mostly dealing with hydrology and paleoclimatic topics.
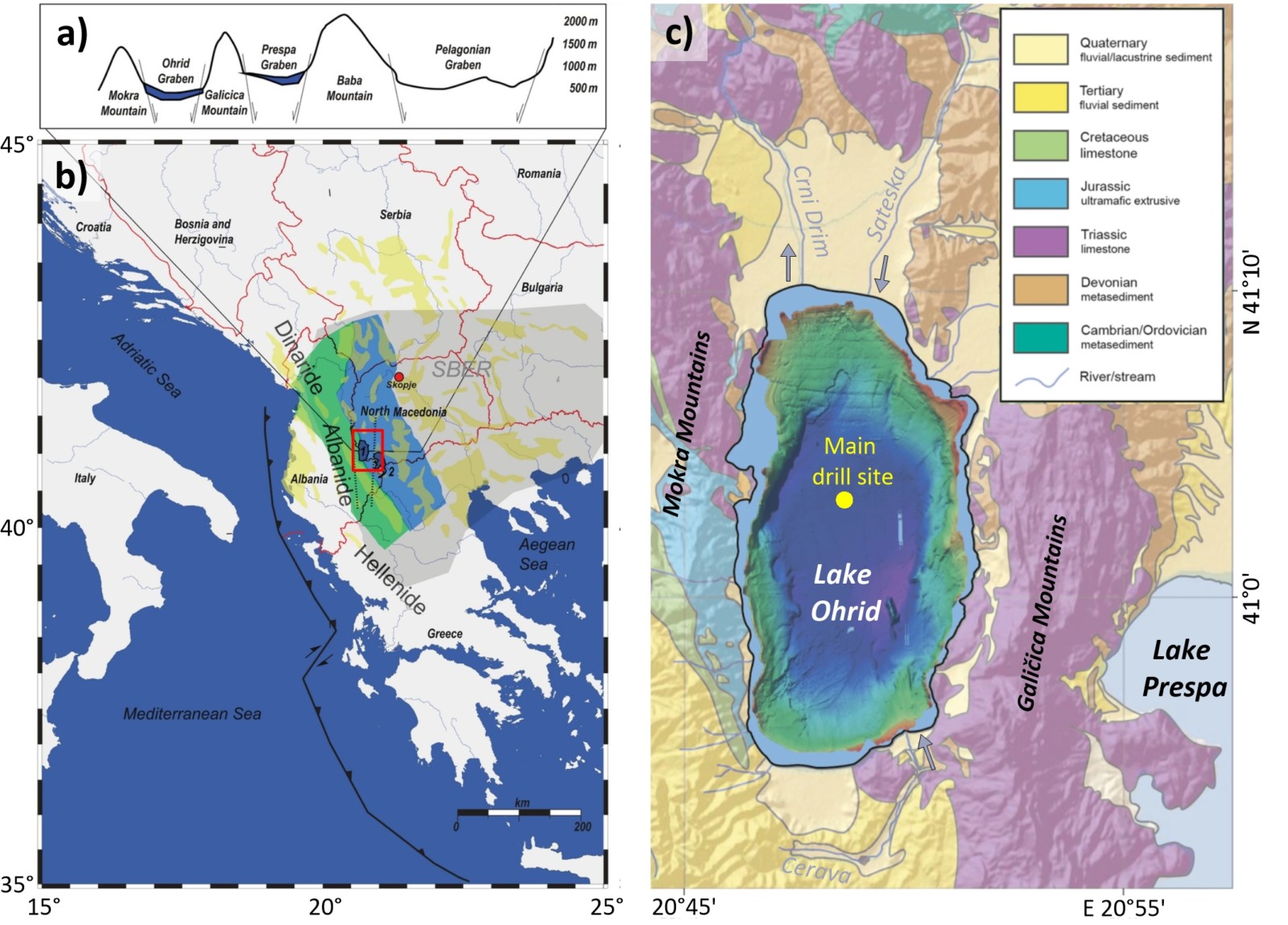
Figure 4: Location of Lake Ohrid. a) Profile from west to east through the graben system. The Ohrid Basin is the youngest of these basins and is still active today. b) Overview of the Balkan Peninsula with the location of Lake Ohrid. The content of the red square is detailed in c) and marks the position of Lake Ohrid (modified after Lindhorst et al., 2015). c) Bathymetric map of Lake Ohrid and geologic map of the surrounding region. The ICDP drill site is highlighted in yellow. Karst water flows through the Galičica Mountains from Lake Prespa into Lake Ohrid (modified from Wagner et al., 2019).
My colleagues and I at the Leibniz Institute for Applied Geophysics were responsible for acquiring/evaluating geophysical downhole logging measurements, specifically with respect to orbital cyclicities. This type of data is acquired after a hole is drilled and sediment cores are pulled. Downhole logging tools are lowered into the now empty hole and measure the physical/chemical properties of the surrounding sediments directly in the borehole. Depending on whether a sediment layer was deposited during a climatic warm or cold phase, it has different properties. Even small fluctuations within a single warm phase can be detected in this way. It is exactly these cyclical changes that we observe and study in the downhole logging data. Compared to core data, downhole logging data has the great advantage of being recorded without gaps and, in the case of Lake Ohrid, even with a very good resolution. This is what makes them so convenient for cyclostratigraphic investigations.
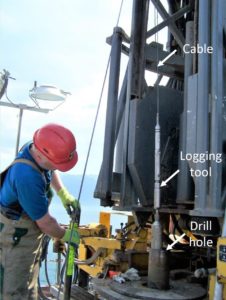
Figure 5: Logging specialist Thomas Grelle from the Leibniz Institute for Applied Geophysics on the drill rig in Lake Ohrid prepares the downhole logging tools. Meanwhile one tool enters the borehole (© Thomas Grelle).
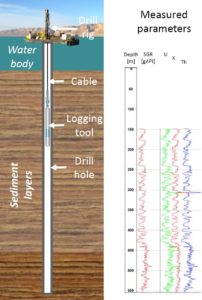
Figure 6: Schematic view of geophysical downhole logging in lake environments. After the drilling operations, logging tools are lowered in the hole. Usually, measurements are performed while the tool is pulled upwards. Precise depth measurements and parameters. In this case spectral gamma radiation (SGR) which is the result of radioactive elements Uranium (U), Potassium (K) and Thorium (Th).
How we found half precession in Europe
We conducted a study using these data in which we also planned to investigate the ‘classical’ Milanković cycles, when a colleague of mine asked: “What are those small regular peaks in your record? Could that be a half-precession signal?” I was curious because, as a PhD student who was quite new in the field of cyclostratigraphy, I had never really heard of half-precession.
And this was only the initial seed for further investigations. We wanted to understand the signal in a broader spatial view. So the search began for other datasets. And these data had to meet several criteria: 1) The data should be continuous and long enough to be comparable to the ~1 Ma record from Lake Ohrid, 2) the proxy of the records should be climate sensitive both in glacials and interglacials, 3) the temporal resolution needs to be able to reflect half-precession, and 4) the data needs to be available.
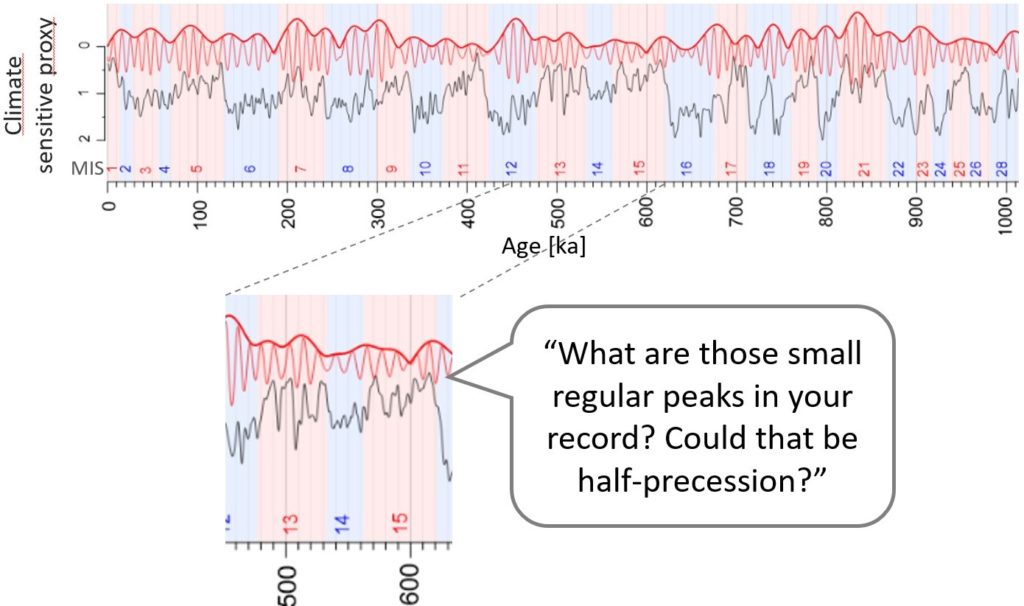
Figure 7: The first appearance of half-precession in our records. In this case a Uranium record from Lake Ohrid in black. The red lines mark different filters of the record. The background colors describe cold (blue) and warm (red) periods.
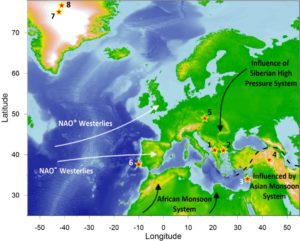
Figure 8: Europe and the prevailing climate systems. The position of the used datasets is indicated by stars. Lake Ohrid is number 1.
Simultaneously we needed to find a way to quantify the half-precession-signal in the different records. We quickly realized that we faced an issue in several records concerning the glacial-interglacial variability. It appeared that climatic signals are mostly only apparent in interglacials. But since the records also contain glacials, in which the half-precession-signal is often very weak, the glacial periods pulled down the overall half-precession-signal. To put it plainly, the half-precession-signal was quite weak in most records. But why did we continue from here despite seeing that the signals are close to the detection limit?
It was because we observed a pattern when we plotted the values on the European map. There was a trend showing that the records in the south(east) had a ‘clearer’ half-precession-signal compared to the northern records. Several publications describe the half-precession as an equatorial signal, but there is still debate going on about this assumption. Did our results support the theory that half-precession is a tropical signal? And if so, why can we observe half-precession in European records?
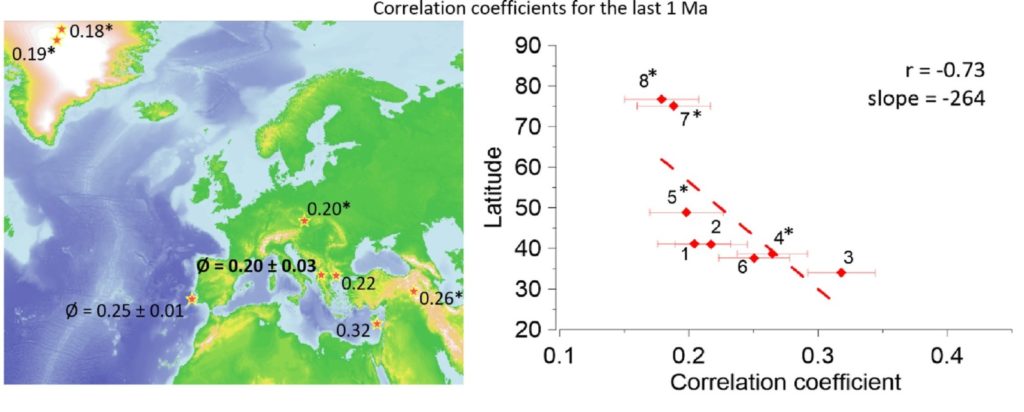
Figure 9: ‘Clarity’ of the half-precession-signal for the last 1 Ma plotted on a map of Europe (left) and in a latitude dependent cross-plot (right). The numbers is the right panel indicate sites as in Fig. 8. The clarity increases from northwest to southeast (modified from Ulfers et al., 2022).
Half-precession as indicator for paleoclimate connectivity
The idea behind the half-precession-signal being present in Europe (or in mid- to high-latitudes in general) is the connection of the different climate zones. Unlike the classical Milanković cycles, which can have a direct influence on the European climate, the half-precession signal first has to find a path from the equator to the high/mid latitudes. This can be explained best using the Nile river as an example. When there is increased monsoon activity in low latitude Africa, the Nile responds to that and transports information about the African subtropics northwards into the Mediterranean. And it is such mechanisms involving ocean-, fluvial- and atmospheric dynamics that can play a major role in transporting the half-precession-signal from the equator to mid- and high latitudes. Following this idea, the half-precession-signal becomes weaker the further one moves north. And that’s what we see in the data. Thus, if we observe an increased half-precession-signal at mid- and high latitudes, this may be an indication of connectivity between climate zones.
More research is needed for a better understanding of this phenomenon. For this purpose it is planned to investigate the origin of the half-precession at the equator in more detail. In sediment cores off the west coast of Africa, climatic changes could be observed based on dust input from the Sahara. Which role the half-precession plays in this dynamic and in which way the signal is propagated into the Atlantic and to Euroep is the subject of the next project on half-precession.
For further information check out the ‘CycloPod’ Podcast by David De Vleeschouwer. In episode 9 we talk about half-precession in Europe:
https://www.cyclostratigraphy.org/podcast
References:
Berger, A., Loutre, M.F., McIntyre, A., 1997. Intertropical Latitudes and Precessional and Half-Precessional Cycles. Science 278, 1476–1478.
Hilgen, F. J., Hinnov, L. A., Aziz, H. A., Abels, H. A., Batenburg, S., Bosmans, J. H., de Boer, B., Hüsing, S. K., Kuiper, K. F., Lourens, L. J., Rivera, T., Tuenter, E., Van de Wal, R. S. W., Wotzlaw, J. & Zeeden, C. (2015). Stratigraphic continuity and fragmentary sedimentation: the success of cyclostratigraphy as part of integrated stratigraphy. Geological Society, London, Special Publications, 404(1), 157-197.
Lindhorst, K., Krastel, S., Reicherter, K., Stipp, M., Wagner, B., Schwenk, T., 2015. Sedimentary and tectonic evolution of Lake Ohrid (Macedonia/Albania). Basin Research 27, 84–101.
Ulfers, A., Zeeden, C., Voigt, S., Abadi, M. S., & Wonik, T. (2022). Half-precession signals in Lake Ohrid (Balkan) and their spatio-temporal relations to climate records from the European realm. Quaternary Science Reviews, 280, 107413.
Wagner, B., Vogel, H., Francke, A., Friedrich, T., Donders, T., Lacey, J.H., Leng, M.J., Regattieri, E., Sadori, L., Wilke, T., Zanchetta, G., Albrecht, C., Bertini, A., Combourieu-Nebout, N., Cvetkoska, A., Giaccio, B., Grazhdani, A., Hauffe, T., Holtvoeth, J., Joannin, S., Jovanovska, E., Just, J., Kouli, K., Kousis, I., Koutsodendris, A., Krastel, S., Lagos, M., Leicher, N., Levkov, Z., Lindhorst, K., Masi, A., Melles, M., Mercuri, A.M., Nomade, S., Nowaczyk, N., Panagiotopoulos, K., Peyron, O., Reed, J.M., Sagnotti, L., Sinopoli, G., Stelbrink, B., Sulpizio, R., Timmermann, A., Tofilovska, S., Torri, P., Wagner-Cremer, F., Wonik, T., Zhang, X., 2019. Mediterranean winter rainfall in phase with African monsoons during the past 1.36 million years. Nature 573, 256–260.