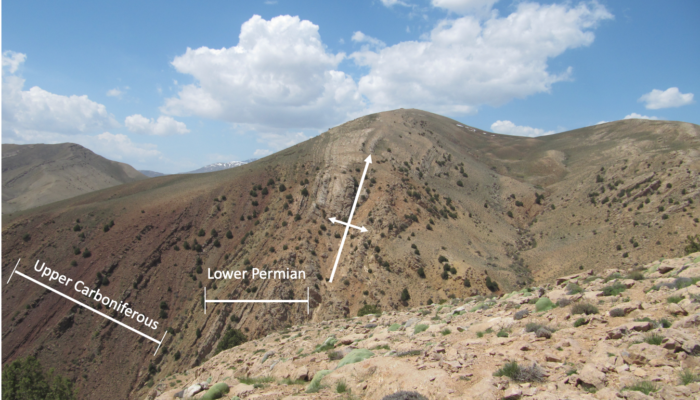
This blog summarizes two recent articles about dust in the Paleozoic.
Planet Earth is presently undergoing a profound climatic turnover. Improved understanding of both the mechanisms contributing to the present climate change and its consequences for the biosphere, including human society, will not only provide the knowledge required to cope with its effects, but may also shed light on the forces that have driven similar events in the past.
The present changes in Earth’s climate have generated a considerable increase in the volume of atmospheric dust.
In the modern world, dust-borne nutrients from the Saharan desert fertilize photosynthetic organisms across the Atlantic Ocean and the distant Amazon rainforest. Recent studies show both that dust was also fueled ecosystem fertilization in the past, and that dust fertilization may have played a role in sequestering atmospheric CO2 around 300 million years ago (Sur et al. 2015; Sardar Abadi et al., 2020).
By increasing levels of limiting nutrients, especially iron, dust enabled primary producers such as marine algae and cyanobacteria to proliferate in the ancient oceans. Higher abundances in primary producers potentially contributed to higher carbon capture, helping to explain declines in atmospheric CO2 around 300 million years ago during the peak of an ancient glacial period.
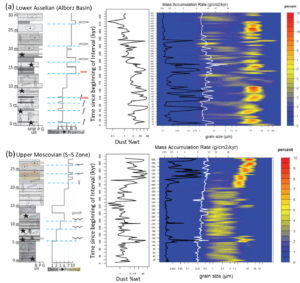
Figure 1: The temporal variation of the dust weight percent and volume grain size distributions through the Lower Permian Emarat Formation (a) and Upper Carboniferous Moscovian Absheni Formation (b).
Atmospheric dust preserved in loess, marine sediments, and ice cores provides valuable insight to past climates of the Cenozoic and especially Pleistocene, but remains little studied for Earth’s deep-time records. Dust and loess deposits are widespread and increasingly recognized and studied in the late Paleozoic record of equatorial Pangaea, but less so for extratropical regions. In a recent study, we used the silicate mineral fraction of carbonate facies as a proxy for eolian dust contributions to the Central Persian Terrain (CPT) along the northeastern margin of Gondwana at ~30o S, and study aspects of this record to reconstruct atmospheric dust loading and dust provenance for parts of the Permo-Carboniferous of this region. The CPT hosted a cyclic succession of warm-water, shallow-marine carbonates from the Middle Pennsylvanian (Carboniferous) to the earliest Permian, and our dataset includes two ~25 m sections from the Moscovian and Asselian, sampled at 20 cm intervals. Bounding surfaces between successive cycles (high-frequency sequences) are recognized by either abrupt basinward shifts in facies, or subtle exposure features; these high-frequency sequences range from 1 – 5 m thickness and are interpreted to record glacioeustatic variations. Time series analysis of the silicate mineral fraction through the studied interval supports the hypothesis of orbital forcing. Dust deposition increased during glacial to relative interglacial periods, with fine dust increasing ~9 fold and coarse dust increasing ~26 fold (Fig. 1). This bimodal distribution may indicate multiple sources—an interpretation consistent with highly variable Strontium isotope values. The most likely sources are within the Arabian–Nubian Shield, with sources shifting between Arabia and Ethiopia during different phases. Dust flux increased during glacials, possibly reflecting glacial gustiness, with provenance changes driven by glacial-interglacial shifts in circulation (Sardar Abadi et al., 2021).
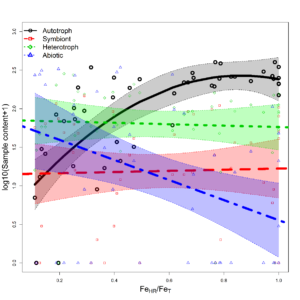
Figure 2: Autotrophic content increases with bioavailable Iron (FeHR/FeT) up to a ratio of 0.6 and then flattens out.
The importance of dust as a source of iron (Fe) for marine primary production in modern oceans is well studied but remains poorly explored for deep time. Vast dust deposits are well recognized from the late Paleozoic, and provisionally implicated to drive biotic production through iron fertilization. We document dust impacts on marine primary productivity in Moscovian (Middle Pennsylvanian, ~307 Ma) and Asselian (Lower Permian, ~295 Ma) carbonate strata from peri-Gondwanan terranes of Iran, at southern mid paleo-latitudes. Interestingly, the fossil community composition of both intervals shifted with bioavailable Iron content (highly reactive iron (FeHR) / total iron (FeT)) in samples as shown by significant correlations between PCoA axis scores and FeHR/FeT (Fig. 2). The results demonstrate that highly reactive Fe borne by dust enhanced microbial and cyanobacterial growth (Fig. 3), thus promoting carbonate precipitation and increasing marine Corg burial. Consequently, dust likely stimulated both organic and inorganic carbon cycling, helping to maintain low pCO2 even as terrestrial repositories for organic carbon (peat/coal) diminished from the Carboniferous through Permian times (Soreghan et al., 2019).
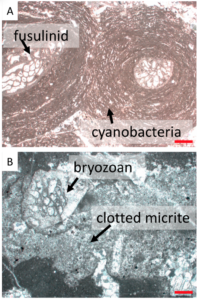
Figure 3: Representative carbonate fossils in the studied intervals. Red scale bars = 0.5 mm. A. cyanobacterial filaments surrounding fusulinids in oncoids within the Lower Permian. B. clotted micrite fabric in association with a bryozoan fragment.
References
Sardar Abadi, M., Soreghan, G.S., Hinnov, L., Heavens, N.G., Gleason, J.D. (2021). Atmospheric dust flux in northeastern Gondwana during the peak of the late Paleozoic ice age. Bulletin, 133(5-6), 992-1006.
Sardar Abadi, M., Owens, J.D., Liu, X., Them, T.R., Cui, X., Heavens, N.G., Soreghan, G.S. (2020). Atmospheric dust stimulated marine primary productivity during Earth’s penultimate icehouse. Geology, 48(3), 247–251.
Soreghan, G.S., Soreghan, M., and Heavens, N.G., 2019, Explosive volcanism as a key driver of the late Paleozoic ice age: Geology, 47(7), 600–604.
Sur, S., Owens, J.D., Soreghan, G.S., Lyons, T.W., Raiswell, R., Heavens, N.G., Mahowald, N.M. (2015). Extreme eolian delivery of reactive iron to late Paleozoic icehouse seas. Geology, 43(12), 1099–1102.