Tonga Volcano Eruption 2022-01-15 (image was produced by Anna Perttu - Volcanic Risk Solutions, Massey University, Palmerston North, New Zealand)
Jelle Assink, Senior Geophysicist at KNMI, takes us through the details of the various kinds of waves produced by the Hunga eruption in Tonga earlier this year…
On January 15, 2022, a powerful volcanic eruption occurred in the Tonga archipelago in the Pacific Ocean when the submarine Hunga volcano exploded around 04:15 (UTC). This explosion marked the climactic end of an eruptive phase that started on 19 December 2021, after several years of quiescence. Never before have atmospheric waves from such an explosion been recorded so extensively on digital sensor networks on the Earth’s surface as well as from space. Based on the amplitude measurements of the pressure wave that circumnavigated the globe several times, it has been estimated that the explosion was comparable in size to that of the 1883 Krakatau eruption.
In this blog we will further discuss some of the atmospheric and seismic observations following the climactic event. A more detailed account on this event is published in the July 2022 issue of Science (Matoza et al., 2022). The study was written by a global research team of 76 authors (from 17 countries!) with expertise in seismology, acoustics, tsunamis, ionospheric perturbations, and volcanology.
Introduction
Hunga is part of the highly active volcanic arc of the Tonga-Kermadec Islands, a subduction zone stretching from the northeast of New Zealand up to Fiji. The volcano is mostly submerged with the exception of the two islands of Hunga-Tonga and Hunga-Ha’apai which are part of the caldera. During an earlier phase in 2014-2015, the two islands were connected, but since the explosion on January 15, they have been separated again.
The January 2022 eruption has had a disruptive effect on people and society, even far away from the volcano. The ash plume, which even reached into the mesosphere, partly precipitated on the surrounding islands. The displacement of water as a result of the volcanic eruption generated tsunamis that caused damage, especially in Tonga. In addition, fast-traveling tsunamis generated elsewhere in the Pacific Ocean, the Caribbean and the Mediterranean. These were driven by the atmospheric pressure wave associated with the climactic eruption (Kubota, 2022). The explosion is the largest since the eruption of the Philippine volcano Pinatubo in 1991. Unlike the eruption of Pinatubo however, the climatic effects of this eruption have been expected to be small.
Pressure wave observed from space
The most prominent atmospheric wave that was detected is the so-called Lamb wave. This type of wave, named after Sir Horace Lamb who described them in 1917, has been observed following large explosions associated with volcanic eruptions (Reed, 1987), meteor air bursts (Brown et al., 2013) and megaton nuclear tests (Donn et al., 1963). The pressure amplitude of the Lamb wave is largest near the Earth’s surface and decreases quasi-exponentially with height. Its propagation speed (approximately 315 m/s), is determined by the temperature and wind in the lower regions of the atmosphere. The wave propagates efficiently because of the reduced geometrical spreading (since it is a surface wave) and low intrinsic absorption rates. Similar to acoustic waves, Lamb waves propagate as an alternation of dilatations and compressions of air. The vibration time of this type of wave can range from several to tens of minutes. Because the air volume displacements are extremely large, gravity also has an effect on the propagation. Therefore, the Lamb wave is called an ‘acoustic-gravity’ wave.
The Lamb wave has been observed by several geostationary weather satellites that provide a continuous view of the Earth’s atmosphere. The geostationary weather satellites can estimate atmospheric temperature by measuring infrared radiation. The Lamb wave’s pressure variations lead to small deviations of a few tenths of a degree Celcius that the satellites are sensitive to. Essentially, the temperature rises due to the compression of air, similar to when pumping air into a bicycle tire.
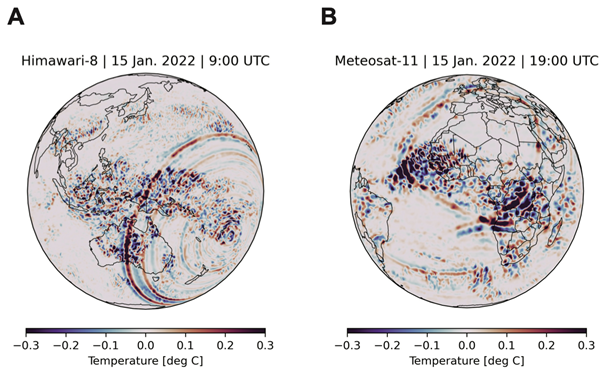
Figure 1: Low-pass filtered satellite imagery from the geostationary (A) Himawari-8 and (B) Meteosat-11 satellites on 15 January 2022. The imagery shows the Lamb wave passing through the atmosphere as small temperature perturbations. Satellite data courtesy of Dr. Jan Fokke Meirink (KNMI).
Images from the Japanese Himawari-8 satellite (Figure 1A) clearly show waves spreading in circles from the point where the eruption took place. The imagery has been low-pass filtered to accentuate the wave. Tracing the wavefront in time indeed corresponds to the expected propagation speed of 315 m/s. On the other side of the Earth, Meteosat-11 imagery (Figure 1B) shows converging wavefronts near the antipode, i.e., the point on the Earth’s surface that is directly opposite to the source location. In the case of the Hunga explosion, the antipode was located in Southern Algeria.
The Science study reports on the observation of at least four minor-arc Lamb wave passages and three major-arc (antipodal) passages, which is similar to the eruption of Krakatau in 1883 (Figure 2).
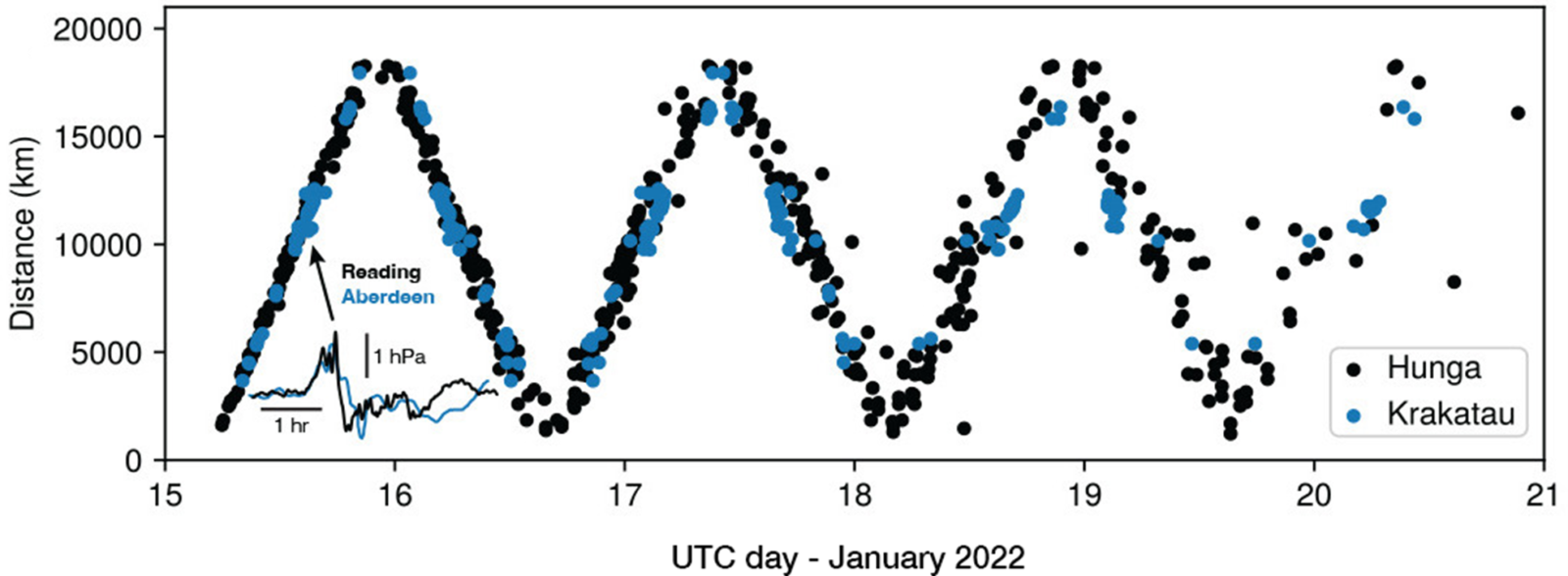
Figure 2: Comparison of Lamb wave arrival times following the 2022 Hunga (black) and 1883 Krakatau (blue) eruptions. A detailed waveform comparison of the pressure wave is shown in the inset. From Matoza et al., Science, 2022. Reprinted with permission from AAAS.
Ground-based observations and seismo-acoustic coupling
In Utrecht, The Netherlands (16,500 km distance), the first Lamb wave arrival was detected around 19:00 UTC as a sinusoidal signal with an amplitude of about 1.5 hPa in the 0.1-1.0 mHz band on (micro)barometers that are part of the network of the Royal Netherlands Meteorological Institute (KNMI). Elsewhere in Europe, similar observations were made around this time.
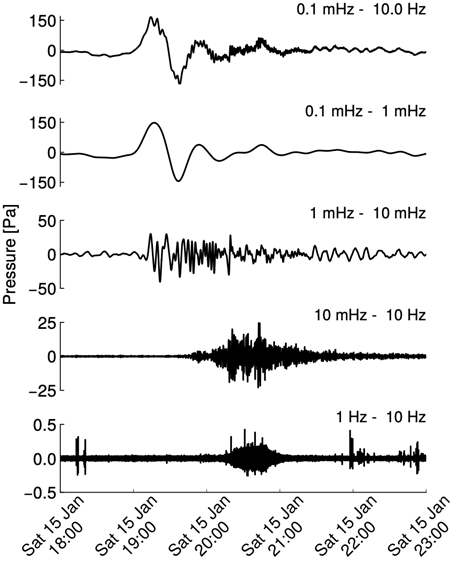
Figure 3: Microbarometer recording at the Royal Netherlands Meteorological Institute (KNMI), De Bilt, The Netherlands of the first pressure wave arrivals in various frequency bands. The complex waveform is composed of various modal waveforms that have been guided in the atmosphere.
Following the Lamb wave arrival, the pressure timeseries reveal additional small-amplitude arrivals with higher frequency content (Figures 3 and 4). This characteristic dispersion is well-known from earlier studies of large explosions, and can be interpreted as a linear superposition of various modal waveforms (Pierce and Posey, 1971). The wavetrain culminates in the detection of broadband infrasound on the microbarometer, with a frequency content of up to 10 Hz (Figure 4; top row). Such signals are unique for signals originating from the other side of the world. In Alaska (~10,000 km distance), even audible signals were detected and reported.
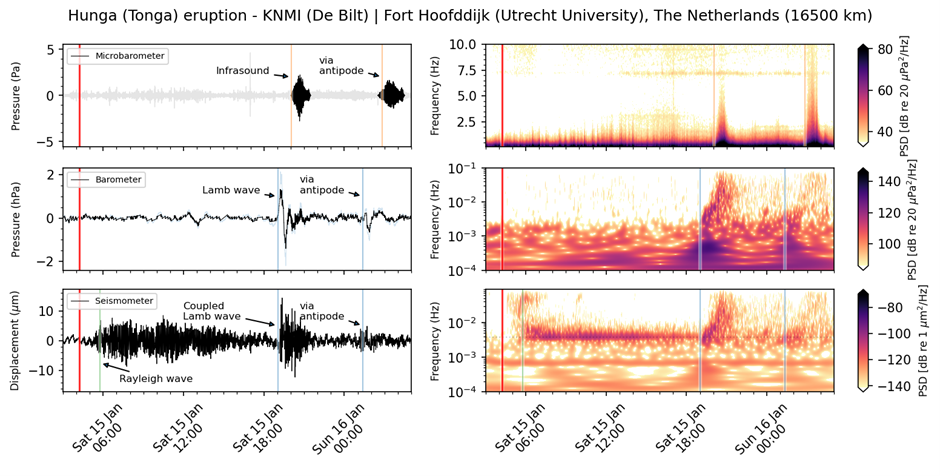
Figure 4: Observations of seismic and pressure waves following the Hunga eruption on 15 January 2022 near Utrecht, the Netherlands. The various features are indicated in the band-pass filtered waveforms (left), along with the corresponding spectrograms (right). Seismic data courtesy of Dr. Hanneke Paulssen (Utrecht University).
In addition to the pressure waves, the volcanic eruption also generated vibrations that traveled through the earth and were measured on seismometers worldwide. Some of these waves travel through the Earth’s interior, but most of the energy propagates along the Earth’s surface as Rayleigh waves, at a speed of about 3 km/s. The first Rayleigh wave arrival at NARS seismometer NE05 at Utrecht University was detected around 6:00 UTC (Figure 4; bottom row).
Another interesting feature is the coupling of the Lamb wave to the subsurface around the time the Lamb wave passes over the seismometer. It is well known that long-period (i.e., periods longer than 10 seconds) atmospheric pressure waves can contribute to horizontal and vertical displacements that can be measured on seismometers (Sorrells, 1971). Indeed, the characteristic chirp that is visible in the barometer’s spectrogram is also observed in the seismic vertical displacement spectrogram (Figure 4; bottom row).
About six hours later, a smaller antipodal Lamb wave arrival was detected as well (Figure 4; left column). The waveform shows a clear 90 degree phase shift and, similar to the first arrival, also is associated with the detection of high-frequency infrasound and a detectable vertical seismic displacement. Subsequent Lamb wave arrivals (up to a total of six) were detected with even smaller amplitudes.
Further Reading:
Brown, P. G., Assink, J. D., Astiz, L., Blaauw, R., Boslough, M. B., Borovička, J., Brachet, N., Brown, D., Campbell-Brown, M., Ceranna, L., Cooke, W., De Groot-Hedlin, C., Drob, D. P., Edwards, W., Evers, L. G., Garces, M., Gill, J., Hedlin, M., Kingery, A., … Krzeminski, Z. (2013). A 500-kiloton airburst over Chelyabinsk and an enhanced hazard from small impactors. Nature, 503(7475), 238–241. https://doi.org/10.1038/nature12741
Donn, W. L., Pfeffer, R. L., & Ewing, M. (1963). Propagation of air waves from nuclear explosions. Science, 139(3552), 307–317. https://doi.org/10.1126/science.139.3552.307
Kubota, T., Saito, T., & Nishida, K. (2022). Global fast-traveling tsunamis driven by atmospheric Lamb waves on the 2022 Tonga eruption. Science, eabo4364.
Matoza, R. et al., (2022). Atmospheric waves and global seismoacoustic observations of the January 2022 Hunga eruption, Tonga. Science. https://doi.org/10.1126/science.abo7063
Sorrells, G. G. (1971). A Preliminary Investigation into the Relationship between Long‐Period Seismic Noise and Local Fluctuations in the Atmospheric Pressure Field. Geophysical Journal of the Royal Astronomical Society, 26(1–4), 71–82. https://doi.org/10.1111/j.1365-246X.1971.tb03383.x
Pierce, A. D., & Posey, J. W. (1971). Theory of the Excitation and Propagation of Lamb’s Atmospheric Edge Mode from Nuclear Explosions. Geophysical Journal of the Royal Astronomical Society, 26(1–4), 341–368. https://doi.org/10.1111/j.1365-246X.1971.tb03406.x
Reed, J. W. (1987). Air-pressure waves from Mount St. Helens Eruptions. Journal of Geophysical Research-Atmospheres, 92(D10), 11979–11992. https://doi.org/doi:10.1029/JD092iD10p11979
Geoffrey whellams
Is there any recoded effect of harmonic action effecting other volcanic sites ?