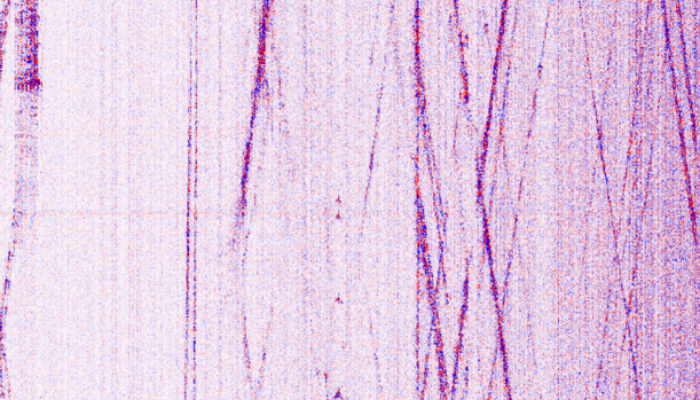
What is Distributed Acoustic Sensing (DAS) and why is it exciting?
Any observational seismologist would agree that our understanding of subsurface structure and dynamics is in great part limited by our ability to acquire data at the right locations, with the necessary spatial and temporal resolution. However, although our ultimate objective would be to cover the Earth with high-quality, broadband seismometers that can recover seismic events ranging from microseismicity, through man-made vibrations to large earthquakes, such an undertaking would be extremely expensive. Even for temporary experiments, the deployment of hundreds of individual sensors is challenging, and investigations end up being limited in both spatial and temporal coverage. In addition, seismic deployments in areas such as urban environments are generally very restricted, despite the importance of characterizing subsurface structure for seismic and other hazard evaluation.
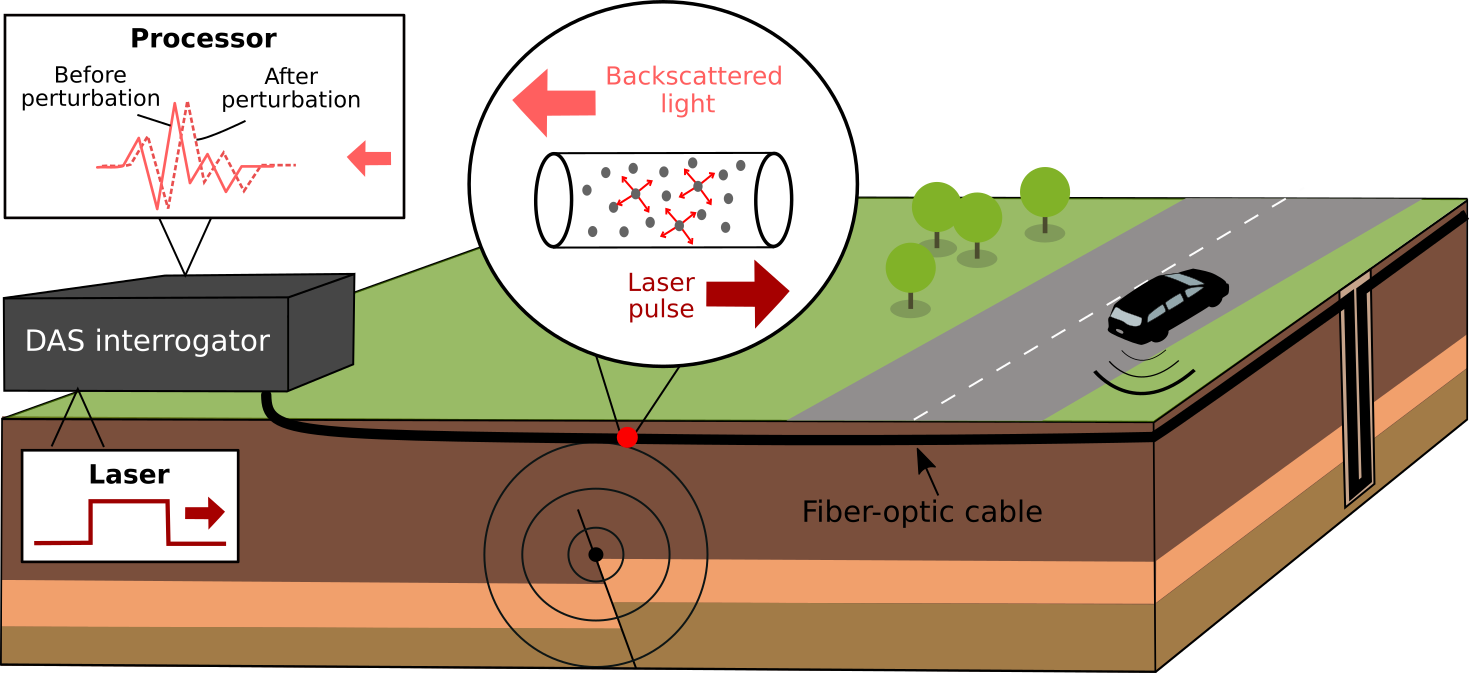
Figure 1. Schematic of DAS. DAS exploits changes in the phase of light that is backscattered from intrinsic impurities in the core of fiber-optic cables. DAS interrogator units are connected to one end of a fiber-optic cable and set up to shoot laser pulses down the fiber-optic cable thousands of times a second. Part of the light pulsed down the cable is scattered at the fiber impurities and recorded back at the interrogator. When a seismic wave passes through the cable, compression or extension is induced, which will cause a change in the phase of the backscattered light. The interferometric system in the DAS interrogator analyses this difference and converts it into longitudinal strain-rate along the axial direction of the cable (Posey et al., 2000; Hartog et al, 2017).
In this increasing need for cost-effective, high-resolution seismic data acquisition, Distributed Acoustic Sensing (DAS) offers an attractive alternative to conventional seismic deployments. In a nut-shell, DAS technology transforms commercial fiber-optic cables into massive arrays of seismic sensors. Fundamentally, DAS exploits changes in the phase of light that is backscattered from intrinsic impurities in the core of fiber-optic cables. In particular, DAS exploits Rayleigh backscattering which, at seismic frequencies, is mostly sensitive to the changes in dynamic strain (strain-rate) caused by the fiber’s motion. DAS interrogator units, as they are usually referred to, are connected to one end of a fiber-optic cable and set up to shoot laser pulses down the fiber-optic cable thousands of times a second. Part of the light pulsed down the cable is scattered at the fiber impurities and recorded back at the interrogator. When a seismic wave passes through the cable, compression or extension is induced, which will cause a change in the phase of the backscattered light. The interferometric system in the DAS interrogator analyses this difference and converts it into longitudinal strain-rate along the axial direction of the cable (Posey et al., 2000; Hartog et al, 2017). Currently, DAS can interrogate fiber-optic cables as long as 40-50 km, with measurement points every few meters at frequencies that range from the mHz to the kHz. In this way, these regular cables are converted into arrays of 10’s of thousands of single-component seismic sensors, providing the opportunity to sample the seismic wavefield at unprecedented resolution at local to regional scales.
Applications in seismology
DAS has been known to the oil and gas industry since the early 90’s, and original applications focused on exploration seismics (Daley et al., 2013; Mateeva, 2013). In the last 5 years, however, there has been a significant growth in the number of studies using DAS for other seismology-related applications, covering a broad spectrum including earthquake seismology (Lindsey et al., 2017, Wang et al, 2018, Yu et al., 2019), geotechnical characterization using ambient noise (Dou et al., 2017), induced seismicity monitoring (Lellouch et al. 2020), glaciology (Walter et al., 2020; Booth et al., 2020), amongst others (Zhan, 2020).
In addition to using dedicated fiber arrays, recent studies have shown that DAS can also be deployed on pre-existing telecommunication fiber networks that are not being used for data transfer purposes (Jousset et al., 2018; Ajo-Franklin et al., 2019). These networks, known as dark fiber, enable leveraging existing infrastructure for gathering high-resolution seismological data (e.g. Figures 2 and 3). This option opens up extraordinary opportunities to perform seismic investigations in challenging environments such as densely populated areas (Spica et al., 2020), where these unused networks are abundant, and even the ocean floor, where DAS recordings of earthquakes and the ambient noise fields are being used to image fault structures and to investigate ocean wave dynamics (Lindsey et al., 2019, Sladen et al., 2019, Williams et al., 2019).
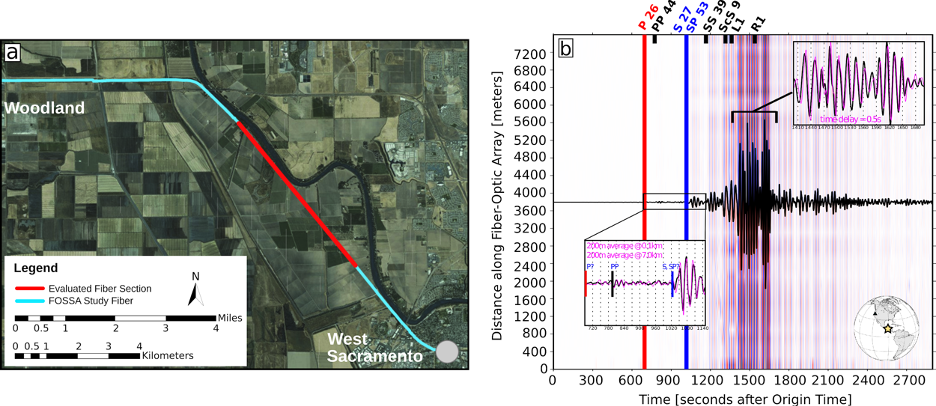
Figure 2. Fiber-Optic Sacramento Seismic Array (FOSSA) experiment, performed along a section of a dark fiber network running from West Sacramento, CA, to Woodland, CA (Ajo-Franklin et al., 2019). (a) Fiber-optic cable path showing section of the cable evaluated in Ajo-Franklin et al. (2019) for ambient noise analysis and earthquake detection. (b) DAS recording of the M8.1 Chiapas (Mexico) earthquake occurred on September 8th, 2017. Black trace shows seismic data for one location along the fiber; red and blue show data for all locations between 0 and 7.6 km along the array. Top right inset = surface waves arriving at the south (black) and north (pink) end of the array; bottom left inset = body waves arriving at the same time at both locations. [Modified after Ajo-Franklin et al., 2019].
The versatility and exciting opportunities offered by this novel sensing technology is increasingly attracting the attention of the geophysical community. This fact is demonstrated by a flourishing number of DAS-focused events in a variety of geophysics conferences and publications. For example, workshops and sessions dedicated to discussing DAS instrument advancements and applications have been and are being organized at the AGU Fall Meeting, the EGU General Assembly, the Seismological Society of America (SSA) Annual Meeting and the Society of Exploration Geophysics (SEG) Annual Meeting. An AGU monograph on DAS is underway, and EGU’s Solid Earth open-access journal is preparing a special issue on Fiber-optic sensing in Earth Sciences. Moreover, the American National Science Foundation has recently funded a Research Coordination Network that aims to engage and facilitate communication with seismologists, hydrologists, volcanologists, cryosphere scientists, and the broad geophysical community by way of workshops, short courses, tutorials, etc.
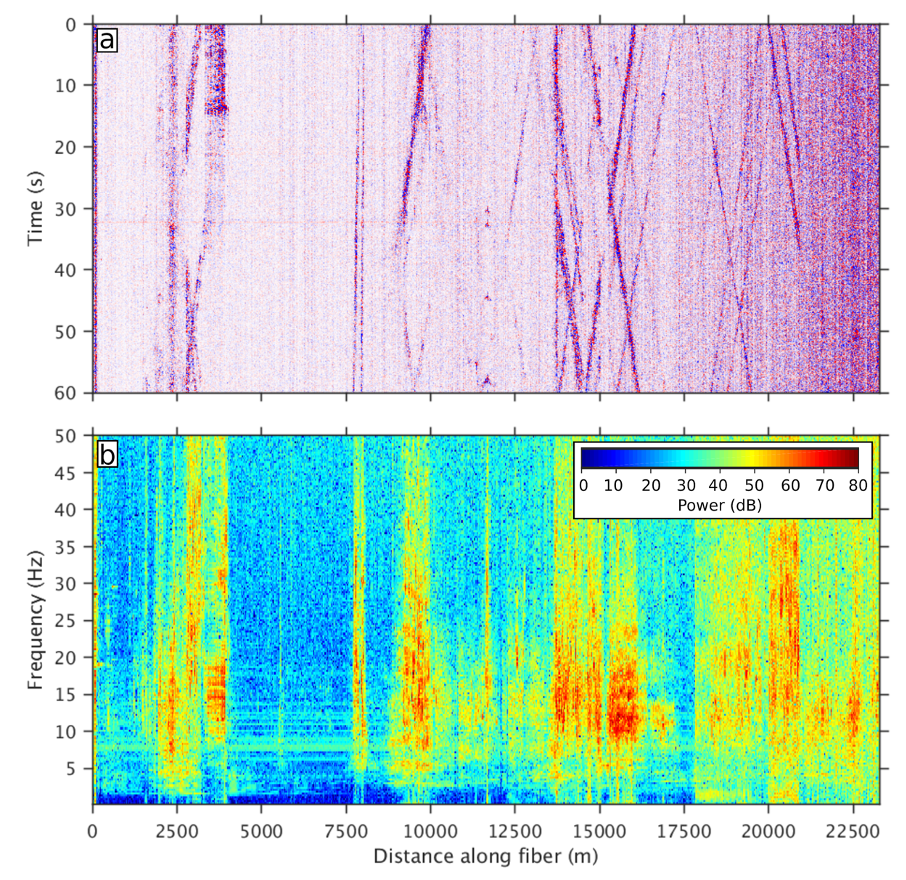
Figure 3. Ambient noise recording along the 23 km-long dark fiber profile of the FOSSA experiment (Ajo-Franklin et al., 2019). (a) 1-minute raw data recording along profile. Signals of vehicles travelling along the roads surrounding the profile are clearly observed. (b) Spectral power of 1-minute ambient noise recording in (a). Most power is concentrated at frequencies between ~ 2 Hz and 30 Hz, corresponding to the infrastructure frequency band. Train-generated surface waves recorded along this DAS array, however, showed high power at frequencies as low as 1 Hz (Rodríguez Tribaldos et al., 2019).
Current challenges and future opportunities
Despite the transformative opportunities provided by DAS arrays, there are still many challenges that need to be addressed in order to fully exploit this new type of sensing. One of the most pressing issues is the large volume of data generated by these arrays. For example, the DAS experiment described in Ajo-Franklin et al. (2019) continuously acquired passive seismic data on a 23 km-long cable with spatial sampling of 2 m at 500 Hz for over 200 days, generating more than 200 TB of data at a rate of ~ 1TB/day. However, new processing algorithms that can leverage high-performance computing (Dong et al. 2020), as well as innovative machine learning approaches (Shiloh et al., 2019), are being developed to address these difficulties. An additional question that is especially important for the seismological community is achieving a full understanding of the DAS instrument’s response. Recent and ongoing studies aim to empirically characterize these systems for a variety of broadband frequencies and deployment modes, and offer encouraging results that help to shed light on the complexities of this novel seismic sensing technique (Lindsey et al., 2020). Another avenue of current research is new fiber-optic cable design and construction, to improve sensitivity and light backscattering as well as to recover multicomponent measurements (as opposed to being restricted to strain-rate along the cable). As a rapidly developing and emerging sensing technology, DAS promises to revolutionize seismic acquisition and expand our ability to understand the Earth’s subsurface and how we interact with it.
References
Ajo-Franklin, J. B., S. Dou, N. J. Lindsey, I. Monga, C. Tracy, M. Robertson, V. Rodriguez Tribaldos, C. Ulrich, B. Freifeld, T. Daley, et al. (2019). Distributed acoustic sensing using dark fiber for near-surface characterization and broadband seismic event detection, Scientific Reports, 9 (1328) doi: 10.1038/S41598-018-36675-8.
Booth, A. D., Christoffersen, P., Schoonman, C., Clarke, A., Hubbard, B., Law, R., Doyle, S.H., Chudley, T.R., Chalari, A. (2020). Distributed Acoustic Sensing of seismic properties in a borehole drilled on a fastowing Greenlandic outlet glacier. Geophysical Research Letters, 47, e2020GL088148. https://doi.org/10.1029/2020GL088148
Daley, T. M., Freifeld, B. M., Ajo-Franklin, J., Dou, S., Pevzner, R., Shulakova, V., Kashikar, S., Miller, D. E., Goetz, J., Henninges, J., Lueth, S. (2013). Field testing of fiber-optic distributed acoustic sensing (DAS) for subsurface seismic monitoring. The Leading Edge, (June). https://doi.org/S0016-6480(10)00349-7
Dong, B., Rodríguez Tribaldos, V., Xing, X., Byna, S., Ajo-FRanklin, J.B., Wu, K., (2020) DASSA: Parallel DAS Data Storage and Analysis for Subsurface Event Detection, IEEE International Parallel and Distributed Processing Symposium (IPDPS). DOI 10.1109/IPDPS47924.2020.00035
Dou, S., Lindsey, N., Wagner, A. M., Daley, T. M., Freifeld, B., Robertson, M., Peterson, J., Ulrich, C., Martin, E.R., Ajo-Franklin, J.B. (2017). Distributed Acoustic Sensing for Seismic Monitoring of the Near Surface: A Traffic-Noise Interferometry Case Study. Scientific Reports, 7(1), 1–12. https://doi.org/10.1038/s41598-017-11986-4
Hartog, A. H., Rao, Y.-J., Ran, Z.-L., Gong, Y., Güemes, A., & Sierra Perez, J. (2017). An Introduction to
Distributed Optical Fibre Sensors. (A. H. Hartog, Ed.). Boca Raton, Florida: Taylor and Francis Group.
Jousset, P., T. Reinsch, T. Ryberg, H. Blanck, A. Clarke, R. Aghayev, G. P. Hersir, J. Henninges, M. Weber, and C. M. Krawczyk (2018). Dynamic strain determination using fibre-optic cables allows imaging of seismological and structural features, Nature Communications, 9(2509), doi: 10.1038/s41467-018-04860-y.
Lellouch, A., Lindsey, N. J., Ellsworth, W. L., Biondi, B. L. (2020). Comparison between Distributed Acoustic Sensing and Geophones: Downhole Microseismic Monitoring of the FORGE Geothermal Experiment, Seismological Research Letters, XX, 1–13, doi:10.1785/0220200149
Lindsey, N. J., Martin, E. R., Dreger, D. S., Freifeld, B., Cole, S., James, S. R., et al. (2017). Fiber-Optic Network Observations of Earthquake Wavefields. Geophysical Research Letters, 44(23), 11,792- 11,799. https://doi.org/10.1002/2017GL075722
Lindsey, N., C. Dawe, and J. Ajo-Franklin (2019). Illuminating seafloor faults and ocean dynamics with dark fiber distributed acoustic sensing. Science, 366, 1103-1107
Lindsey N.J., Rademacher, H., Ajo-Franklin, J.B. (2020) On the Broadband Instrument Response of Fiber-Optic Arrays, Journal of Geophysical Research: Solid Earth, 125, e2019JB018145. https://doi.org/10.
Mateeva, A., Lopez, J., Mestayer, J., Wills, P., Cox, B., Kiyashchenko, D., Yang, Z., Berlang, W., Detomo, R., Grandi, S. (2013). Distributed acoustic sensing for reservoir monitoring with VSP. The Leading Edge,
(October), 3–7. Retrieved from http://library.seg.org/doi/10.1190/INT-2014-0248.1
Posey, R, Johnson,G., and Vohra, S. (2000). Strain sensing based on coherent Rayleigh scattering in an optical fibre. Electronics Letters, 36(20), 1688–1689.
Rodríguez Tribaldos, V., Ajo-Franklin, J.B., Dou, S., Lindsey, N.J., Ulrich, C., Robertson, M., Freifeld, B., Daley, T., Monga, I., Tracy, C., (2019). Surface Wave Imaging using Distributed Acoustic Sensing Deployed on Dark Fiber: Moving Beyond High Frequency Noise, Earth Arxiv, February 8 https://doi.org/10.31223/osf.io/jb2na
Shiloh, L., Eyal, A., Giryes, R., (2019). Efficient Processing of Distributed Acoustic Sensing Data Using a Deep Learning Approach, Journal of Lightwave Technology, 37 (18), 4755–4762, doi:10.1109/jlt.2019.2919713
Sladen A., Rivet, D., Ampuero, J.P., De Barros, L., Hello, Y., Calbris G., Lamare P., (2019). Distributed sensing of earthquakes and ocean-solid Earth interactions on seafloor telecom cables, Nature Communications, 10 (5777).
Spica, Z.J., Perton, M., Martin, E.R., Beroza, G.C., Biondi, B., (2020), Urban Seismic Site Characterization by Fiber‐Optic Seismology, Journal of Geophysical Research: Solid Earth, 125 (3), https://doi.org/10.1029/2019JB018656
Walter F., Gräff D., Paitz, P., Köpfli, M., Chmiel, M., Fichtner, A. (2020). Distributed acoustic sensing of microseismic sources and wave propagation in glaciated terrain, Nature Communications, 11 (2436)
Wang, H. F., X. Zeng, D. E. Miller, D. Fratta, K. L. Feigl, C. H. Thurber, and R. J. Mellors (2018). Ground motion response to an ML 4.3 earthquake using co-located distributed acoustic sensing and seismometer arrays, Geophysics Journal International, 213 (3) 2020–2036, doi: 10.1093/gji/ggy102.
Williams, E., Fernández-Ruiz, M.R., Magalhaes, R., Vanthillo, R., Zhan, Z., González-Herráez, M., Martins, H.F. (2019) Distributed sensing of microseisms and teleseisms with submarine dark fibers. Nature Communications, 10 (5778).
Yu, C., Z. Zhan, N. J. Lindsey, J. B. Ajo-Franklin, and M. Robertson (2019). The potential of DAS in teleseismic studies: Insights from the goldstone experiment, Geophysical Research Letters, 46 (3), 1320–1328, doi: 10.1029/2018GL081195.
Zhan, Z. (2020), Distributed Acoustic Sensing Turns Fiber-Optic Cables into Sensitive Seismic Antennas, Seismological Research Letters, 91(1), 1-15. https://doi.org/10.1785/0220190112
This blog post was written by Guest Author Verónica Rodríguez Tribaldos
(with helpful comments from Nate J. Lindsey and Jonathan Ajo-Franklin)
and revisions from ECS representative Walid Ben Mansour.