Sea ice brine sampling is always great fun, but sometimes somewhat challenging ! As sea water freezes to form sea ice, salts in the water are rejected from the ice and concentrate in pockets of very salty water, which are entrapped within the sea ice. These pockets are known as “brines”. Scientists sample these brines to measure the physical and bio-geochemical properties, such as: tem ...[Read More]
Image of the Week – For each tonne of CO2 emitted, Arctic sea ice shrinks by 3m² in summer
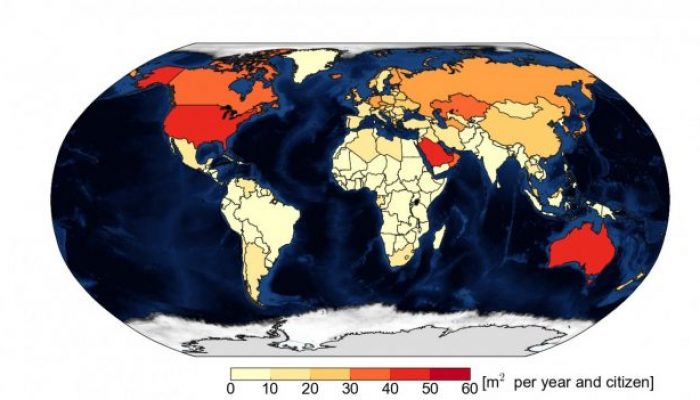
Declining sea ice in the Arctic is definitely one of the most iconic consequences of climate change. In a study recently published in Science, Dirk Notz and Julienne Stroeve find a linear relationship between carbon dioxide (CO2) emissions and loss of Arctic sea-ice area in summer. Our image of this week is based on these results and shows the area of September Arctic sea ice lost per inhabitant d ...[Read More]
Image of the Week: Atmospheric CO2 from ice cores
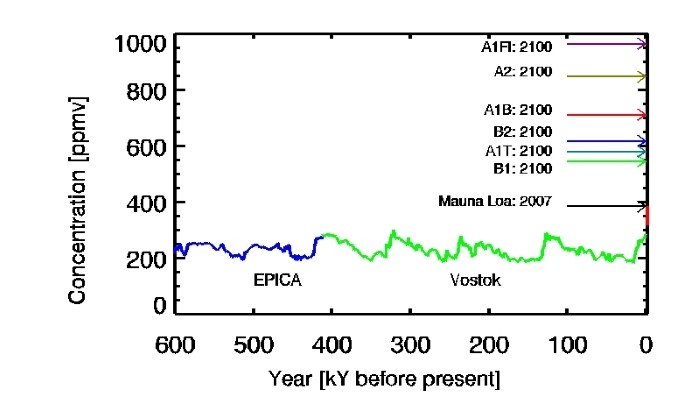
The measurements of atmospheric CO2 levels at Manu Loa, Hawaii read 401.01ppm on the 7th of December this year. To understand the significance of this number, you just need to look at the figure above from the 4th IPCC report. It shows the changes in CO2 concentrations during the past 800,000 years based on ice core measurements. Values have fluctuated between 190ppm and 280ppm. In other words, bo ...[Read More]
Camping on the Svalbard coast
In early April 2015, a small team of 2 Belgian and 2 French researchers went to Svalbard. The goal? Testing new methods to measure sea-ice thickness and ice algal biomass, but also measuring greenhouse gases in the sea ice in relation with the ‘STeP’ (Storfjorden Polynya multidisciplinary study) campaign. With funding from the French Polar Institute (IPEV) and IPSL and logistical arrangements by t ...[Read More]
Do Beers Go Stale in the Arctic? – Jakob Sievers
A story about CO2 -fluxes between sea-ice and the atmosphere What’s it all about? Whenever I have had to describe my PhD research project to people outside of my research community, I have always found it useful to use an analogy most people are familiar with, namely beers. Now that I have the full attention of the entire class, allow me to explain. Say you were to find yourself at an outside café ...[Read More]